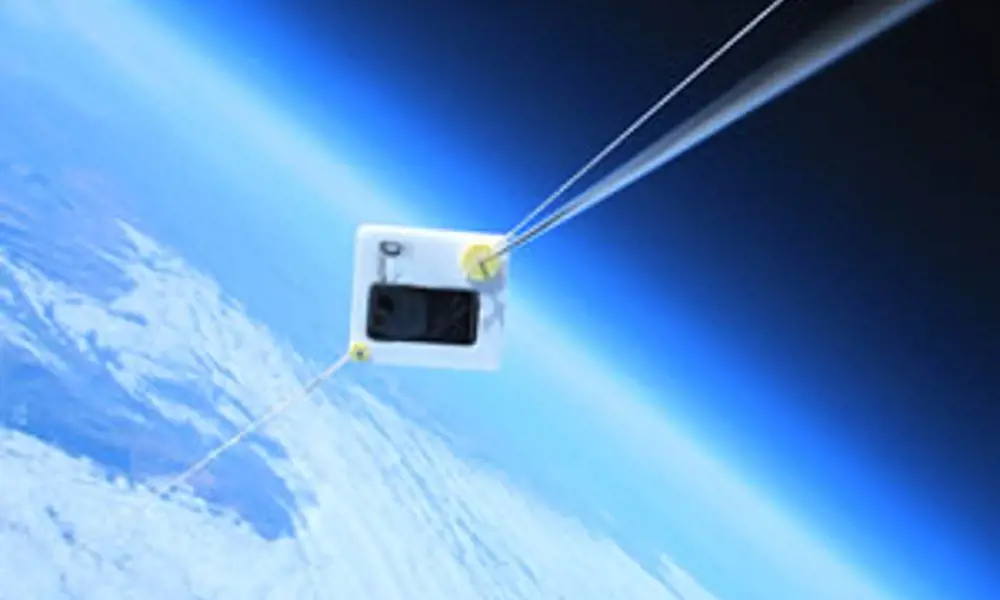
Creating stratospheric instrument platforms
Two test flights, ASTRA 7 and 8, in early 2011 demonstrated the feasibility of using a low-powered, lightweight commodity device (an HTC Trophy smartphone running Windows Phone 7 in this case) as a data logger, communications link and a portal to high performance computing resources in the computational cloud – through Windows Azure. The ASTRA 7 vehicle took this ‘self-portrait’ at an altitude of 18km above South Wales on 4 March 2011. The light blue band above the horizon, giving way abruptly to a much darker region, illustrates the fact that ASTRA 7 had, by this point, left over 90% of the air mass surrounding the planet behind it
The Atmospheric Science through Robotic Aircraft (ASTRA) initiative at the University of Southampton develops technologies for the low-cost exploration of Earth’s atmosphere. Lead investigator Dr András Sóbester outlines how ASTRA is providing atmospheric scientists with a new generation of high-altitude observational platforms.
Many branches of science depend on accurate observation of the physical and chemical parameters of the atmosphere. These include routine weather forecasting, predicting future climate, observing volcanic plumes, and understanding extreme weather, and more complex tasks, for example, monitoring the dispersion of pollutants (such as the fallout from nuclear incidents) and mapping the interaction of atmospheric flows with the topographical features of a landscape. Building an accurate picture of the physics of the atmosphere requires the deployment of instruments that are able to access a wide range of altitudes.
The staple tool of such missions has been the venerable radiosonde, a basic instrument package that rises into the stratosphere suspended under a latex weather balloon inflated with helium or hydrogen. Along the way, this draws up a temperature, pressure, humidity, wind and sometimes ozone concentration profile of the atmosphere. As the balloon and its payload ascend into gradually thinning air, the volume of the lifting gas increases until, eventually, the balloon bursts, usually at an altitude between 20 km and 35 km. The sonde then begins a long fall back to the ground, slowed by a small parachute.
Although easy to deploy, the radiosonde suffers from a number of shortcomings. For example, it cannot be recovered at the end of its flight – its instruments, circuitry and batteries simply become pollutants, unless they are retrieved and handed in by members of the public. For the same reason, it is unsuitable for sample-collecting missions. It is also wasteful as a carrier of more expensive instrumentation and the amount of data it can gather is limited by the bandwidth of its telemetry (communications) system. Additionally, its nearly linear trajectory means that it can only provide a one-dimensional profile of the parameters being measured.
The ASTRA initiative was set up to develop technologies for addressing these shortcomings and for designing a generic, trackable, retrievable, high-altitude balloon launch capability. Low cost, flexibility, customisation and short response times are the key items on the ASTRA design brief.

To enable prototyping, the atom is printed
Smartphones in the stratosphere
Research missions requiring more complex, recoverable instrumentation than the staple, single-use sensors of a radiosonde result in expensive bespoke platforms, which demand a long lead time for development. One cost-cutting measure being investigated by ASTRA is the use, wherever possible, of commodity components and subsystems. On a high-altitude balloon system where tracking, communications, data logging and preliminary processing are indispensable services, a smartphone is an obvious candidate. It offers all of the above elements in a lightweight, compact, energy-efficient, mature and reliable package. In early 2011 the decision was therefore made to design an experimental balloon platform around a smartphone. In March 2011, we at the ASTRA project launched and, a few hours later that same day, successfully recovered our first two phone-based prototype payloads.

It is then fitted with .NET Gadgeteer modules
Thanks to carefully designed foam enclosures, which had been precision-cut on a computer-controlled laser cutter, the Windows Phone 7 handsets survived their stratospheric rides (one of them was still operating perfectly when it was found several hours after landing), during which the pods were exposed to temperatures as low as -64°C. At an altitude of 20 km, their maximum distance from the surface, the phones were in the space equivalent zone – with ambient air pressure down to around 50 mbar.
The phones ran a specially developed app which enabled data logging, communications, and tracking. While they were within the range of 3G masts, they transmitted trajectory data to a remote, ground-based cloud computing service running in Microsoft’s Dublin data centre. This enabled us to carry out calculations that far exceeded the processing capabilities of the phone.
In these test flights the data stream consisted simply of the geographical coordinates and altitude of the payload. On receipt of each sent coordinate set, the flight simulation that computes the new predicted landing site was re-run by the cloud service (see Flight path prediction).

Finally the atom is assembled, ready for flight
The ground crew used the ‘tracking’ mode of the same app running on their phones to follow the progress of the flights, thus expediting the eventual recovery of the payloads. The successful deployment of the ground chase app on ordinary smartphones indicates, perhaps, the potential for a future crowdsourcing model, where the public can get involved in the payload recovery process.
Self-recovering payloads
There is a concept of high-altitude balloon operations that promises even lower costs than the trackable, recoverable platform: a platform that, following balloon burst, flies itself home. This is essentially a high-altitude platform fitted with wings, control surfaces and an autopilot, effectively turning it into a simple, lightweight, small glider that has the capability of guiding itself towards a designated collection point.
The summer of 2011 saw the completion of the prototype of the system and the first phase of its testing. The aircraft containing the sensor package in its forward fuselage is attached to the balloon in a nose-down attitude through a cable that engages with a release mechanism attached to the aft fuselage of the aircraft. Once the autopilot senses that the target release altitude has been reached or the balloon has burst, it commands the opening of the release mechanism and the glider begins its return flight towards the pre-programmed destination.
The aircraft’s modular nature allows the integration of a wide range of instruments, as well as a range of different missions. For example, different types of wings can be fitted to an airframe, depending on the target altitude and the necessary endurance in a particular altitude band – the greater the altitude and required endurance, the greater the wing area required.
This ‘plug-and-fly’ philosophy is enabled by the development of low-cost wing and fuselage rapid manufacturing technologies which build on years of unmanned air systems experience at the University of Southampton. Extensive use of laser-cut foam parts and rapid prototyping technologies (see Rapid prototyping) is also driving significant airframe weight savings, while keeping costs down.
Where next?
In the basic mode of balloon-launched glider operations, the aircraft merely serves to return the instruments after they have performed their measurements during the ascent. There is, however, potential in the system for more sophisticated missions.
A block of airspace, for example, could be examined to map the variation of the concentration of an aerosol cloud. ‘To map’ in this context would mean building a statistical model that, for any given latitude, longitude and altitude within the block would yield an estimate of the required parameter. There are many formulations for such a model – neural networks, Gaussian process models and polynomial models are a few of the more commonly used techniques. They all have one requirement in common, if their accuracy is to be uniformly high. They can only fill in (interpolate) the gaps efficiently between the actual observations if these observations fill the target block uniformly. This is a tall order for a conventional balloon-borne platform, as its ascent usually draws a nearly straight line across the target airspace block – but a gliding descent could be more efficient.
If the glider or a fleet of gliders were launched from a balloon or a fleet of balloons then this would increase the sampling efficiency. The payoffs are potentially significant. Releasing instrumented, auto-piloted gliders could reduce the numbers required by at least a factor of five, while also ensuring that even these can be collected and reused – a significant saving considering that the cost of a single dropsonde is the same as that of a mid-range laptop.
More intriguingly still, could we engineer a fleet of gliders such that, depending on what they each find on their exploratory descent, they could potentially act as a swarm drawn towards the more promising parts of the block being explored?
In addition to the technical challenges, developing such systems involves tackling regulatory issues. Currently, the operation of self-recovering gliding sondes is limited to special, segregated blocks of airspace in the UK. One of the goals of the ASTRA team is to work closely with the Civil Aviation Authority on the better airspace integration of such systems.
These are some of the challenges being tackled currently by the ASTRA team, in close collaboration with its science partners. One such institution is the British Antarctic Survey, with whom we are currently designing a system suitable for measurements in atmospheric mountain waves in the South Atlantic. We are also collaborating with the Met Office’s research units at Cardington and Camborne on the evaluation and testing of rapid prototyped platforms – potential application areas for these include weather research, as well as the operational forecasting that forms the basis of the forecasts issued to the public.
The ultimate goal is to introduce a range of new tools to atmospheric science, which, by virtue of their low operating cost, can make both routine operations and complex research campaigns more affordable or considerably more extensive.
Keep up-to-date with Ingenia for free
SubscribeOther content from Ingenia
Quick read
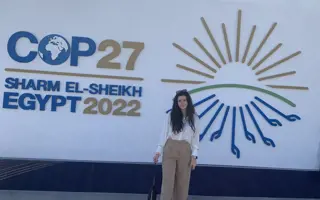
- Environment & sustainability
- Opinion
A young engineer’s perspective on the good, the bad and the ugly of COP27

- Environment & sustainability
- Issue 95
How do we pay for net zero technologies?
Quick read
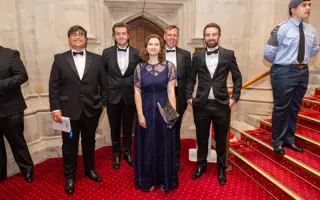
- Transport
- Mechanical
- How I got here
Electrifying trains and STEMAZING outreach
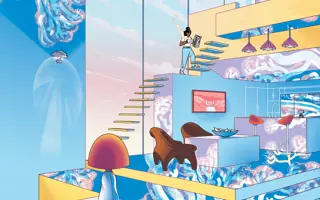
- Civil & structural
- Environment & sustainability
- Issue 95