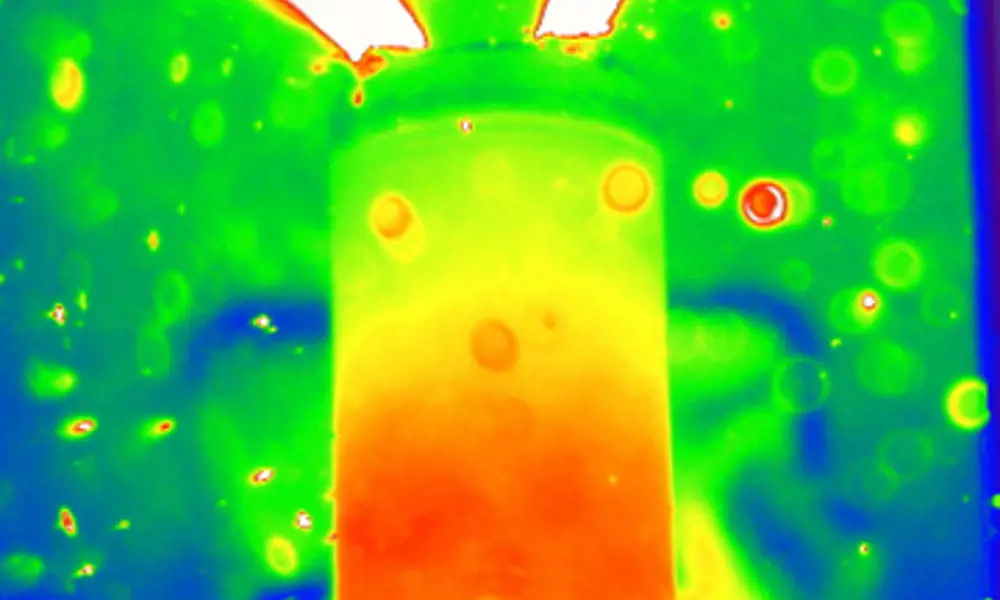
Lithium-ion batteries
A lithium-ion (Li-ion) battery is assembled from three basic components: an anode, cathode and electrolyte. During charging, Li+ ions are moved by an electrochemical driving force from the cathode to the anode through the medium of the ionically conducting electrolyte. During discharge, the reverse happens, and as the Li+ ions move, the coupled transport of an electron to ensure charge neutrality is able to perform useful work. The quantity of Li-ions transferred indicates the total amount of energy available within the cell, and the rate of transfer indicates its power.
There are a variety of cathode materials available commercially that provide the ‘source’ of lithium within the cell. Nearly all commercially available Li-ion batteries use a graphite anode material, and as the battery is charged, lithium is removed from the cathode material, conducted through the electrolyte and ‘intercalated’ (inserted) between the graphene galleries in the characteristically layered graphite structure. This process is remarkably reversible, and the Columbic efficiency for state-of-the-art Li-ion cells can be around 0.9999. This high efficiency guarantees the long cycle life of Li-ion cells compared to alternative battery chemistries.
This high efficiency guarantees the long cycle life of Li-ion cells compared to alternative battery chemistries
However, these cells are not without fault: ‘capacity fade’ is a common and frustrating experience, as overextended cycle-life battery capacity drops and batteries need increasingly frequent charging. While these durability issues remain a concern, it is the more rapid and dangerous failures that have recently made headlines.
The constituent materials in a cell work best within a safe operating window that defines the safe temperature and voltage range, as well as the maximum current that the cell can accept during charging (and deliver during discharge).
Trying to charge a cell too quickly can lead to the precipitation of metallic lithium within the cell, whereas voltages outside the safe operating window can lead to degradation of the electrolyte with the possibility of gas generation. External effects can also influence how the cell behaves, and extremes of temperature and pressure are known to have safety implications.
Increasingly sophisticated battery management systems are designed to protect the batteries from these extremes
Increasingly sophisticated battery management systems are designed to protect the batteries from these extremes; when you plug your mobile phone in to charge, control systems monitor the current and voltage ‘seen’ by the cell and keep it within its comfort zone.
If something does go wrong, there is also hardware inside cells to mitigate against failure. This includes separator materials that prevent short circuiting, positive temperature co-efficient devices that limit cell voltage at elevated temperatures, and pressure relief vents that trigger in the event of excess gas generation to prevent cell rupture.
Catastrophic failures include thermal runaway events, which can occur when heat generated within a cell triggers an exothermic reaction, releasing more heat and driving further exothermic reactions. When the rate of heat generation exceeds the rate of rejection, the thermal runaway proceeds, which can lead to fires and explosions.
Catastrophic failures include thermal runaway events, which can occur when heat generated within a cell triggers an exothermic reaction, releasing more heat and driving further exothermic reactions
In very rare occurrences, manufacturing defects have also been known to cause cell failure. Li-ion cell electrodes are manufactured in extremely high volumes, and the electrodes are assembled in tightly packed configurations to maximise volumetric energy density. While the quality control processes are robust, contaminants in the process can cause problems; for example, any metallic contaminants can puncture the separator and cause short circuiting between the electrodes. Similarly welding, assembly and electrochemical ‘formation’ are subject to highly stringent quality controls, as they can also jeopardise cell safety if left unchecked.
The drive to maximise volumetric energy density and miniaturise cells must be tempered with safety guarantees.
Thankfully, failure rates are estimated to be as low as one failure per 40 million cells; it is therefore highly unlikely that you will ever experience a cell failure firsthand. However, with the increasing presence of these products, and their requirement to operate in a range of environments, scientists and engineers are tackling the challenge of designing safer and more durable batteries, both for Li-ion cells and next-generation energy storage technologies.
***
This article has been adapted from "How does that work? Lithium-ion batteries", which originally appeared in the print edition of Ingenia 69 (December 2016).
Contributors
Dr Paul Shearing is a Chair in Sustainable Energy Engineering and Director of The ZERO Institute at the University of Oxford. He is a Royal Academy of Engineering Chair in emerging battery technologies. At the time of publication, he was a Professor in Chemical Engineering at UCL (University College London), where research interests covered a broad range of electrochemical engineering themes including battery safety
Keep up-to-date with Ingenia for free
SubscribeRelated content
Electricals & electronics
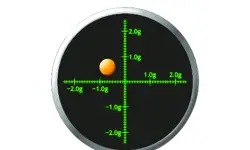
Accelerometers
Used in earthquake measurements, laptops, planes and even in stargazing apps, today’s accelerometers are much smaller than when they were first developed in 1927. Find out how they detect movement and vibration.
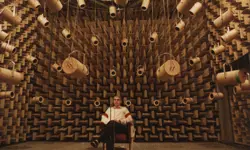
How to maximise loudspeaker quality
Ingenia asked Dr Jack Oclee-Brown, Head of Acoustics at KEF Audio, to outline the considerations that audio engineers need to make when developing high-quality speakers.
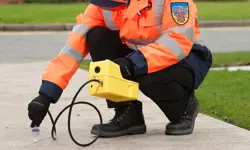
Cable fault locator
The winner of the Institute of Engineering and Technology’s 2014 Innovation Award was EA Technology’s CableSnifferTM, which uses a probe and chemical sensing technology to identify faults, saving energy companies millions of pounds each year.
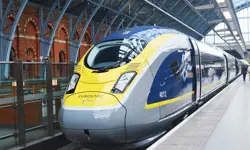
High speed evolution
In December 2010, Eurostar International Ltd awarded a contract for 10 new high speed trains to Siemens. The company has used a system developed over decades to maximise the performance and passenger-carrying ability of its 320km/h trains.
Other content from Ingenia
Quick read
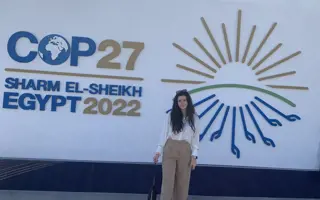
- Environment & sustainability
- Opinion
A young engineer’s perspective on the good, the bad and the ugly of COP27

- Environment & sustainability
- Issue 95
How do we pay for net zero technologies?
Quick read
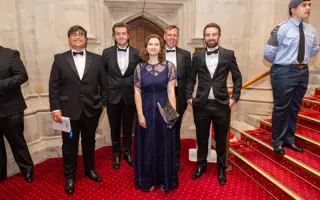
- Transport
- Mechanical
- How I got here
Electrifying trains and STEMAZING outreach
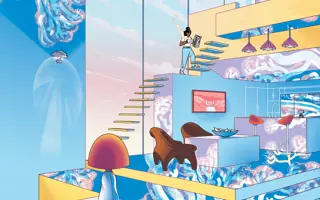
- Civil & structural
- Environment & sustainability
- Issue 95