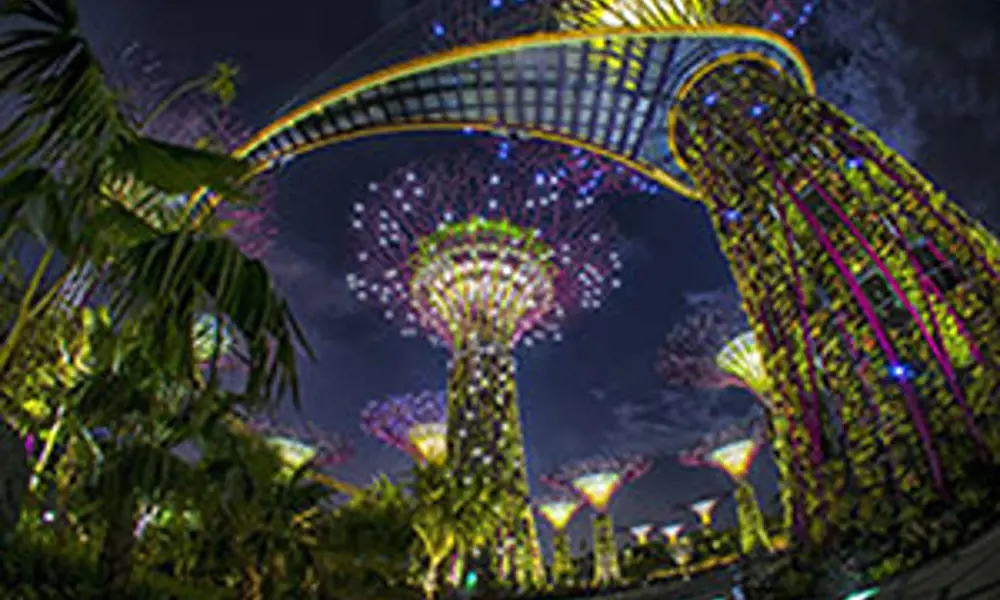
Singapore's Supertrees
RIBA’s Lubetkin Prize is awarded annually to the architects of the best new building outside the European Union. It was won in 2013 by Wilkinson Eyre and Grant Associates for Cooled Conservatories, Gardens by the Bay in Singapore. Within the gardens are 18 vertical gardens known as ‘Supertrees’, which perform a multitude of functions, including planting, shading and working as environmental engines for the gardens. It also won the CIBSE 2014 International Project of the Year for Atelier Ten © Darren Chin
Behind the eye-catching new gardens on Singapore’s waterfront, created by a team of UK engineers and architects, lies a combination of measures that hold lessons for the sustainable design of buildings in hot and humid conditions. The team devised innovative techniques to ensure the conservatory air conditioning remained carbon-neutral, winning RIBA’s 2013 Lubetkin Prize. Ingenia talked to Patrick Bellew RDI FREng, of environmental engineers Atelier Ten, who outlined the engineering challenges and solutions generated by the Gardens by the Bay project.
Gardens by the Bay is the centrepiece of a new financial centre being created on reclaimed land in the Marina Bay area of Singapore. Its 58 hectare site includes two steel‑framed domes between 38m and 58m in height, enclosing a combined area of 20,000m², making them some of the largest greenhouses in the world. The Flower Dome recreates Mediterranean springtime conditions with mild, dry days and cool nights, while the Cloud Forest Dome represents mountainous tropical regions with high humidity but relatively cool temperatures.
From the outset, the project’s greatest challenges were to deal with the balance between the daylight required for the plants to flourish and the intense solar gain on the equator, while finding a way to cool and dehumidify Singapore’s hot and humid air for the domes without imposing a large carbon footprint. The UK is known internationally for its expertise in reproducing environments for non-native plant species to grow, such as in London’s Kew Gardens and the Eden Project in Cornwall. But while those projects keep hothouse plants warm in a cool climate, it was a greater challenge to cool plants in a subtropical climate in an energy‑efficient manner.

Part of the answer was in the creation of the garden’s most distinctive features – the concrete-cored, steel-clad ‘Supertrees’, some nearly 50m tall. Covered in plants, these structures provide sustainable energy while simultaneously providing the infrastructure supporting the site’s enormous climate‑controlled conservatories.
City in a Garden
Gardens by the Bay is part of the Singapore government’s vision of transforming the sovereign city-state into a ‘city in a garden’. It chose Grant Associates of Bath to lead the £350 million Bay South project, Wilkinson Eyre as the principal architects and Atelier One and Atelier Ten – both based in London – serving as, respectively, the structural engineers and the environmental and building services engineers. The project emphasises sustainability and demonstrates environmental responsibility.
Early plans were to harness solar power to provide the energy needed to cool and dehumidify Singapore’s hot and humid air. But Singapore, although hot, is also very cloudy, reducing efficiency for most of the day and potentially overstressing the system when the sun does come out. Conversations with the National Parks Board (NParks) of Singapore led to a work able solution.

Chilled water pipes being installed within the pathways of the Flower Dome, to absorb some of the solar radiation and so reduce the load on the displacement air supply system
The discussions showed that NParks was maintaining and pruning several million trees in metropolitan Singapore, including sending tonnes of tree cuttings a week out of the city, mostly to landfill or for incineration. So a 7.2MW biomass boiler was developed to burn the horticultural waste. To reduce the moisture content to the optimum level, it was decided to mix this with waste packing cases, conveniently available from the nearby freight terminal. Steam from the boiler now feeds a turbine that delivers electricity to the site’s energy centre – with any surplus fed into the grid. The high-temperature water downstream of the turbine drives absorption chillers, which generate chilled water.
Once cleaned, the resulting exhaust gases from the biomass furnaces needed to be released from a very high level, necessitating the use of chimneys. In response, the team devised the plant-covered Supertrees around the chimney flues. The design team used a number of ‘passive’ and ‘active’ measures to further minimise energy use: passive to design the domes to optimise daylight and minimise unnecessary heat gain and active to design the environmental systems to treat and distribute the air as effectively as possible – see Sustainable Supertrees.
Sustainable Supertrees

As well as holding ferns, vines, orchids and also a large collection of bromeliads, the Supertrees are fitted with environmental technologies that mimic the ecological function of trees. There are photovoltaic cells that capture solar energy which can be used for functions such as lighting and the collection of rainwater, used for irrigation and fountain displays. They also serve as air intake and exhaust functions, part of the conservatories’ cooling systems with an elevated walkway between two of the larger Supertrees © Craig Shepherd
At the heart of the gardens are the huge Supertrees designed by Grant Associates, 18 of them between 25m and 50m high and grouped in three clusters. These provide an armature for ducts, flues, shafts and pipes to help remove all evidence of engineering services from the skyline. They also provide shade during the day and dramatic lighting at night. They support photovoltaic solar panels laid (almost) flat on their tops, and vertical planting panels on their sides. A slender aerial walkway is suspended between them via wire rope cables, appearing to float unsupported 22m above the ground, and the largest Supertree supports a two-storey bar and viewing gallery accessed by a lift and twin spiral staircases.
Each tree is supported by cylindrical precast concrete rings, forming a hollow core, cantilevering from the ground and supporting all the environmental devices. The skin is an interconnected array of steel members giving the appearance of branches growing from the ground, fanning out to a broad canopy at the top. The strength is in the shape of the surface.
The light upper canopy is conic in form and is made from a membrane structure in tension stretched between steel elements that are fixed back to the concrete core. The structural frame consists of a double layer lattice that uses the offset between the inner and outer layer to enhance stiffness out of plane. The inner and outer layers are divided into eight panels of identical branch patterns that are rotated half a bay, overlapping to create an apparent randomness across the skin. Stability is then provided by connecting the radials to a series of equally spaced circumferential cables, which complete the anticlastic surface that works together as a whole structure with a high level of structural redundancy.
In time, the true rain forest trees planted in the gardens will reach the same height, and will rival the Supertrees as the greatest wonders of the project.
Desiccant Technology

Flower Dome section, also known as the cool-dry biome. This conservatory is maintained at a temperature and humidity suitable for growing European plants (25°C and 65% relative maximum humidity during the day). Air is introduced for cooling at ground level through integrated slots in the internal structures. Liquid desiccants are used to remove moisture from the air prior to cooling by chilled water. The desiccants are regenerated using a combination of waste heat from a biomass CHP system and solar energy. Additional radiant cooling is incorporated in pathways to absorb incoming solar radiation and return air is drawn from high levels in the space back to the plant in the basement
1. Return air duct to reuse cold air
2. Hot air purged to outside via openings in façade
3. Active shading on exterior of façade
4. Displacement ventilation integrated to planting areas
5. Air intake
6. Air handling plant rooms contain both supply and extract air handling units
7. Underfloor supply duct
8. Fabric cooling to all hard flooring
9. Displacement grilles at regular intervals
10. Motorised mounted impulse fans to ensure air velocity is 0.5 - 1m/s
11. To energy centre
12. Desiccant conditioner to dehumidify air before reaching AHU
Desiccants are commonly found in packaging to keep equipment such as cameras dry before sale. They work by directly removing moisture from air through a chemical process, usually by using the water-absorbent qualities of materials that are composed of salts.
These materials can also be liquids: in effect, very strong solutions of salty water that are so salty they absorb water from air that passes through a spray of these solutions. Removing water from the air in this way results in the air heating up (the reverse of evaporative cooling). But by coupling the salt solution to free cooling from a cooling tower, the vast majority of this heat can be absorbed without additional energy expenditure. This results in a liquid that absorbs water vapour and increases in volume, leaving the air at a similar temperature but drier. When combined with conventional cooling, liquid desiccant technology can deliver air at the required temperature and humidity with less energy than a conventional system.
The chosen liquid desiccant was lithium chloride. Passing the air through a spray of this solution reduces the relative humidity from 90% to 30%, while turning the ‘strong’ desiccant solution to a ‘weak’, or dilute, solution. This weak solution is then passed through a series of regenerator units which remove the water from the solution by treating it with waste heat from the biomass system, and returning it to the strong desiccant tank where it can be reused for dehumidification. The exhaust air from the regenerators is discharged through the Supertrees in their cluster around the lake.
An added benefit of this system is that ‘potential’ energy can be effectively stored in the form of liquid desiccant in storage tanks, to help balance supply and demand.
Reducing energy use
Treating humid air is notoriously energy-intensive: in a conventional system, the air supply would be overcooled by using chilled water to remove the water vapour by condensation, and then reheated to achieve the required temperature. Instead, the design team looked to a technology used in the southern United States to cool industrial buildings such as food stores: desiccant conditioning systems – see Desiccant technology.
For the ‘passive’ measures, the domes had to let in large amounts of light while minimising solar heat gain. The enclosed space of the Flower Dome is 170m by 86m, and 35m high. The Cloud Forest dome is smaller in plan (118m by 77m), but much taller (54m) in order to contain an artificial mountain.
The original concept was for a series of arches comprising solid fins, with single glazing suspended beneath in a simple mullion system. Analysis proved this to be ineffective: the deep structure obscured too much beam radiation and resulted in inadequate daylight on the planted area. There was also too much radiation when the sun was out and directly overhead.
The glazing was a key factor. Single glazing would be cheap and light, but there was no effective way to keep the solar gain down. The surface temperature of the external surface would frequently be below the dew point of the outdoor air resulting in a more or less continual layer of condensation which would shroud the external surface. The answer was to create specialist double-glazed units with a low-emissivity coating on the inner face of the units’ outer sheet of glass to reflect most of the infrared light. The inner sheet is laminated safety glass, with the added advantage that the protective layer of PVB (polyvinyl butyral) within the lamination also reflects most of the ultraviolet light. The units thereby let in about 65% of the incident daylight with only 35% of the solar heat transferred.
The design teams then analysed alternative structures to determine which provided the best balance of daylight and solar control. The optimal solution – and also the most cost-effective – turned out to be a steel grid shell to hold the glazing, tied with hangars to more-slender external steel arches. The grid shell supports itself, while the arches resist wind loading. This allows the grid to be remarkably slender: the ribs of the grid shell have been made as thin as possible, with their edges tapered inwards in section, to minimise the solar-shading.

The Cloud Dome and Flower Dome. Paul Baker of Wilkinson Eyre Architects says: “The complementary yet distinct curved forms of the biomes are generated from the geometry of a hyperbolic curve, and so contain a large volume within a relatively small surface area. Not only does this geometry enable the structure to be optimised (allowing a lightweight, column-free interior), it works well environmentally, creating a stack-effect with warm air drawn up to stratify according to temperature within the space. The gentle forward tilt of the Flower Dome towards Marina Bay also shades the north façade so it never receives the full impact of the sun.” © Craig Shepherd
Architectural challenges
The shape of the shell for each dome was generated from a rotated hyperbolic curve, planned by the structural engineer and architect, with the apex tilted towards the north. The tilting had the beneficial effect that the north walls became near-vertical so that little or no solar gain would enter on this side, thus eliminating the need for external shading, maximising daylight and at the same time saving cost. The shape also introduced asymmetry which required some special engineering measures, such as pre-stressing the arches, particularly for the flatter Flower Dome.
Here, the nose or apex of the hyperbolic surface was attached to the arches and tensioned, effectively pre-stressing the arches to form a rigid boundary. Between this boundary and the ground spans the large south gridshell. The key to this is that the grid shell is braced along the line of the apexes, forming effectively an arched beam at right angles to the arches, and this beam is then tensioned against the arches forming a ‘rigid’ boundary. The north surface, since it is exposed to low wind loads, did not require support from the arches, allowing them to lean forward (away from the glass façade) from the hyperbolic apex down to the ground, forming a cathedral-like promenade along the marina front.
Even with all the measures to maximise sunlight and minimise solar gain, the studies showed that often, on cloudless days with the sun directly overhead, the heat was enough to burn plants and overload the air conditioning system. The domes needed a variable shading system. Shading inside the grid shell would have been easiest, but this would have trapped heat at the highest level within the dome.
The answer was an ingenious system of retractable external shades across all but the northern faces of the two domes. Some 400 triangular polyester sailcloth shades are rolled up and completely concealed within the lower section of the steel arches when not in use, and are actively controlled to deploy by cable when required to modulate internal daylight levels to the desired level. An intelligent self‑learning algorithm controls each shade individually, adjusting the shading to match the conditions at the time.
The introduction of the shades required another inspirational piece of engineering. To maintain the blinds’ cable system and motors, it is necessary to access the whole external surface. The novel solution is a building maintenance unit that can travel all the way over the surface between pairs of arches. A lattice-frame beam, spanning adjacent arches, supports two independent cradles and a crane hook for replacement of glass panels.
This is probably the world's first large-scale biomass-powered environmental system generating power, refrigeration and dehumidification from a single waste stream.
Hot air con
The principal means of air conditioning the domes is a displacement air supply system that delivers dried and cooled air at low level into the occupied zone. Relative densities dictate that the cool air remains below the hot air which limits the volume of the building that needs conditioning, so reducing plant capacity and energy use. It also allows the conditioning air to be delivered at around 18°C (rather than at 12°C which would be the norm for a conventional conditioning system) and this elevated supply temperature results in significant energy savings.
The displacement air is supplemented by the cooling of the pathways and pavements within the domes (by chilled water pipes embedded in the concrete) in order to absorb and remove incident solar radiation. This reduces the amount of heat gain to be dealt with by the air systems, and also improves comfort for visitors.

In the Cloud Forest, looking up at the artificial mountain with its vertiginous aerial walkways. Above the slender grid shell roof, the triangular shades have been deployed to protect the plants from tropical sun © Craig Shepherd
In the Cloud Forest Dome, direct evaporative humidification (misting) is used to provide the very high humidity levels required and also enhances cooling performance.
Determining the necessary air supply and modelling its distribution within the domes was a major challenge. Initial calculations were done using dynamic thermal energy models to predict peak heat gains and mass flow balances to estimate flow rates; these gave the scale of the cooling required. Subsequently, computational fluid dynamics (CFD) modelling was used as an iterative design tool to detail, analyse and optimise the airflow.
The UK Continental Shelf has been producing large amounts of oil and gas since the 1970s. Virtually all of the infrastructure put in place in the North Sea since then will require decommissioning over the next 30 years. Brian Nixon, Chief Executive of Decom North Sea, outlines how the industry plans to do this in one of the harshest of maritime environments.
The early CFD studies showed that the air surrounding the mountain in the Cloud Forest Dome would become hotter than acceptable at high levels with the displacement system alone. As a result, a hybrid ventilation system was developed: air is supplied through displacement terminals at the bottom of the building and at the top of the mountain, but at intermediate levels within the mountain, jet diffusers are used to drive local mixing, thus limiting local stratification. Above the mountain, the air is allowed to stratify (as in a normal displacement system), before being extracted and re-circulated back to the basement plant.
Sustainable credentials
At the start of the project, the Singapore government instructed the design team to endeavour to make the carbon emissions from the conditioning systems no worse than would be experienced in a modern Singapore office building – a major challenge considering the demanding requirements of the plants and the high light levels required. The resulting system ensured that the displaced carbon from onsite renewable energy generation (biomass, supplemented by photovoltaic power) totals 2,050 tonnes of CO ² per year, compared with carbon emissions from the cooling systems for conditioning the domes of 1,770 tonnes of CO ² annually.
This is probably the world’s first large-scale biomass‑powered environmental system generating power, refrigeration and dehumidifi-cation from a single waste stream, and though few future buildings will replicate the Singapore domes, the lessons could have a profound effect on the design of more conventional buildings in hot and humid cities in Asia, the Middle East and elsewhere.
Desiccants are relatively unused and have plenty of potential, particularly where (as with Gardens by the Bay) designers can work with clients to identify waste from outside the site that can be harnessed for energy. Many humid cities have large central cooling plants that simply dump the waste heat, and this could be harnessed to dry desiccants. In cities with a lot of sunshine, solar power could be used for the same purpose.
Certainly, when it was announced that the Gardens by the Bay project had won RIBA’s 2013 Lubetkin Prize, the judges congratulated the winning team for having created greenhouses covering two hectares that were carbon-neutral and for pushing the boundaries not only environmentally but also structurally, giving the city a new and public landmark.
Plant habitat
A large variety of plants will grow naturally and abundantly in Singapore’s tropical climate – including the national flower, the orchid – and these fill much of the Gardens by the Bay. But within the two domes it was necessary to create the cooler (and in one case, drier) environments required by non-native plants – the opposite of the tropical glasshouses of western Europe, and far more difficult to achieve.
Another feature of the gardens is the use of ‘green walls’, both within the domes and outside. These come in three forms: geotextile reinforced-earth walls with planting help to soften steep slopes which would otherwise be concreted; vertical planting panels are used on the sides of the Supertrees and some walls; and ‘living render’ provides a porous and roughly textured concrete surface that incorporates a proportion of organic material in the concrete mix to create moisture retention and rooting zones for epiphytes (‘air plants’).
Biography
Patrick Bellew RDI FREng is founding director of Atelier Ten (1991). He is one of the UK’s Royal Designers and is a Chartered Building Services Engineer with more than 30 years’ experience in the design of high‑performance buildings and systems.
Keep up-to-date with Ingenia for free
SubscribeOther content from Ingenia
Quick read
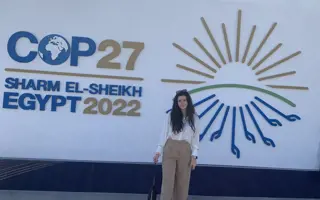
- Environment & sustainability
- Opinion
A young engineer’s perspective on the good, the bad and the ugly of COP27

- Environment & sustainability
- Issue 95
How do we pay for net zero technologies?
Quick read
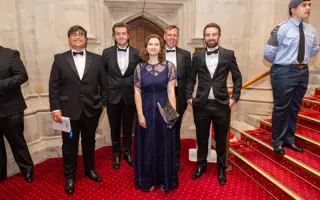
- Transport
- Mechanical
- How I got here
Electrifying trains and STEMAZING outreach
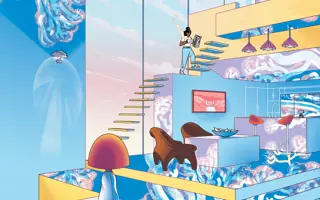
- Civil & structural
- Environment & sustainability
- Issue 95