Small Modular Reactors
The development of small, factory-built nuclear reactors is driven by a need to cut capital costs, provide power away from large distribution networks and reduce greenhouse gas emissions. Professor Richard Clegg and Professor Mamdouh El-Shanawany of Lloyd’s Register look at the recent surge of interest and the technology behind small modular reactors.

The Russian 50 Years of Victory is the largest and most powerful ice breaker constructed to date. It is the first Arktika-class ice breaker to have a spoon-shaped bow that breaks through ice up to 2.5 m and is driven by two nuclear reactors that produce 75,000 hp © Barry Griffiths
As nuclear power generation has become more established, the size of reactors has grown from the 60MWe levels of the 1950s to today’s 1,600MWe giants*. Despite the operating economies of scale that followed this expansion, there are still high capital costs involved in setting up new plants. These costs, twinned with a demand for smaller-scale, more flexible power generation, now see industry considering small reactors that generate less than 300MWe.
These small modular reactors (SMR) could provide power to remote electrical grids in developing countries or be integrated with renewable technologies, such as wind and solar power, to offset variations in energy generation. They could also be constructed in multiples to be brought on line sequentially and used to better manage fluctuations in electricity demand. ‘Modular’ refers to the fact that the reactors are fabricated in an offsite factory and then delivered to site – in some designs already fuelled for final installation.
The latest designs have significantly reduced construction times, building on existing safety features and the emergency responses associated with large-scale nuclear power plants. Their smaller geometries and lower fuel inventories also enhance their ability to be cooled, reducing the likelihood of vessel failure during an adverse event.
Naval propulsion
The concept of small modular reactors isn’t new. Back in the 1950s, proponents of nuclear power imagined a world where tiny nuclear reactors, dubbed nuclear batteries, powered cars, aeroplanes, trains, and even fridges.
While these ideas were never fully realised, small reactors were developed for naval propulsion, with the first nuclear-powered submarine, USS Nautilus, launched in 1955. The 10 MWe nuclear power pack transformed submarines, giving them greater range, faster sustainable speeds of 20 to 25 knots attainable for weeks on end and the ability to stay submerged for longer. Lloyd’s Register estimates that some 700 nuclear reactors have since taken to the water – typically in nuclear-powered submarines, aircraft carriers and ice-breakers – with around 200 currently at sea.
Why now?
The operating economies of scale that came with traditional large nuclear plants are now being overshadowed by the massive capital costs of building one from scratch. The industry trend towards smaller-scale, more flexible power generation, such as wind and solar energy, has led to a revival of small reactors that generate less than 300 MWe. The development of these new SMRs to provide electricity on land is spreading, with a handful of manufacturers including Westinghouse, GE Hitachi, Babcock & Wilcox and Areva designing and looking to build prototypes. These designs are being developed as ready-to-go reactors or integrated into relatively small nuclear power plants. These caravan-sized units could suit countries that lack the robust electrical network needed to transmit the massive output generated from a conventional large nuclear power plant.
As oil and gas exploration focus on ever-more remote regions, the technology could also offer a cheaper alternative to the standalone diesel generators currently used to power fossil fuel extraction. The fuel required by conventional generators to power operations at the Alaskan tar sands projects, for example, is repeatedly shipped in via a tanker or flown in by plane. This is costly, generates carbon emissions and is weather-dependent. Shipping in an SMR that will typically generate power for 12 years without refuelling – be it via truck or train – would bypass these limitations.
Three key advanced SMR reactor designs are currently being considered, based on light water, fast reactor and high temperature gas-cooled technologies – see Examples of SMR designs. While each has its pros and cons, the designs are generally simpler and will be cheaper to mass produce and site than larger versions. Most are designed with a high level of ‘passive safety’, not requiring operator actions or electronic feedback to shut down safely in an emergency. In addition, the American Nuclear Society recently reported that many of the safety features required on large reactors are not necessary for these small designs.
Light water reactors
The US is largely focusing on small, light water reactors, thanks to its large pool of industrial experience in this type of reactor. These are thermal reactors that use normal water (as opposed to deuterium oxide, also known as heavy water) as a coolant and neutron moderator. The reactor design is basically a scaled-down version – less than 200 MWe – of existing US plants, which is likely to help development and, importantly, speed licensing applications in the country.
Recognising the export potential of these SMRs, the US Department of Energy (DoE) in January 2012 called for applications from industry to develop light water reactors. The $452 million shared funding opportunity is a bid to drive SMR development forward. The cost share agreement covers a five-year period and would involve equal spending by the winning team and the government of up to $904 million. The winner or winners will be announced in September 2012, from competitors Westinghouse, Babcock & Wilcox, Holtec and NuScale Nuclear. Each is designing a small modular reactor (SMR) based on a light water reactor.
Holtec and NuScale Power have also signed agreements with the US DoE to build demonstration reactors at the Department’s Savannah River complex. The Holtec reactor will take around two years to construct, with the company hoping to have its first unit up and running by 2018. NuScale Power is operating on a similar timeline. Meanwhile, Russia, Argentina, France, South Korea, China, Japan and South Africa are working on SMRs based on existing light-water reactor designs. The UK also has expertise and experience in SMRs, with the potential to develop its position as both a designer and manufacturer.

NuScale Power has designed a modular, scalable nuclear power plant that can contain up to 12 of its 45 MWe reactors. Each reactor would have its own combined containment vessel, reactor system, and designated turbine-generator set. In this multi-module plant, one unit can be taken out of service without affecting the operation of the others
Fast neutron reactors
The fast neutron reactor is an alternative design that promises to be smaller than light water types. These reactors typically use liquid metals as a coolant, instead of water, allowing ‘fast neutrons’ of higher energies to drive fission in the reactor.
As such, these reactors use the full energy potential of the nuclear fuel, rather than the 1% consumed by light-water reactors, significantly reducing the radioactive lifetime of waste through transmutation and consumption of transuranics – those elements that have a higher atomic number than uranium. This can extend the refuelling interval to as much as 20 years. But they also create a different radioactive species that can present a different set of challenges. The reactors need a fast-reacting safety system, and cannot rely on passive-safety features alone.
Researchers in the US, Korea and Russia are currently working on small, fast neutron reactors. A 70 MWth/25 MWe reactor from US-based Gen4Energy (formerly Hyperion Power Generation) is perhaps the most well-known. The portable, sealed reactor will measure 1.5 m wide and 2.5 m high, contain no moving parts, and could operate for up to a decade without refuelling. A prototype is currently being built alongside the Holtec and NuScale Power designs at the Savannah River demonstration site.
At the same time, researchers at the Argonne National Laboratory in Chicago, USA are developing a larger design, called the Secure Transportable Autonomous Reactor (STAR). The 400 MWth/175 MWe fast neutron reactor contains passive safety features and is designed to operate autonomously for its 15-year fuel cycle.
The US energy giant General Electric and Japanese conglomerate Hitachi have joined forces to construct a fast reactor – the Power Reactor Innovative Small Module (PRISM) – that can actually generate electricity from spent nuclear fuel. GE Hitachi estimates that around 95% of the spent nuclear fuel from conventional light water reactors could be used to generate electricity, and has laid out plans to build a reactor recycling plant in the US. The alliance has also confirmed talks with the UK’s Nuclear Decommissioning Authority to use the technology to deal with the nation’s civil plutonium stockpile.
High temperature gas-cooled reactors
The third SMR design is based on the concept of a high temperature gas-cooled reactor. Classed as a ‘Generation IV’ reactor, and yet to be built, these reactors will have very high outlet temperatures and be suitable to generate process heat for industrial applications or to produce nuclear-assisted hydrogen for fuelling the hydrogen economy, such as for the transport sector.
China-based energy firm, Chinergy, is pioneering one of the most advanced high-temperature gas-cooled SMR projects to date, and has submitted plans to build up to 18 210 MWe reactors, with passive safety systems at its site in Shandong, linked to driving desalination plants. Other designs come from France-based AREVA as well as US-based General Atomics.

PRISM: recycling nuclear fuel. The PRISM (Power Reactor Innovative Small Modular) is GE Hitachi Nuclear Energy’s next generation reactor. The reactor uses liquid sodium as a coolant, instead of water, which allows the neutrons in the reactor to have a higher energy that drives fission of the radioactive elements, or transuranics. The PRISM reactor can consume the transuranics in used nuclear fuel from conventional water-cooled, light water reactors. GE Hitachi has proposed an ‘Advanced Recycling Centre’ based on six PRISM reactors that could extract more than 100 times the energy from uranium compared to the ‘once-through’ fuel cycle employed in conventional nuclear plants. The centre would produce more than 1,800 MWe, which could power nearly 1.5 million homes
Quicker turnarounds
A common advantage of each SMR is that it can be built relatively cheaply in clean conditions and in a factory environment. A 2011 report from the University of Chicago Energy Policy Institute, commissioned by the US DoE, concluded these reactors could significantly mitigate the financial risk associated with full-scale nuclear plant and compete effectively with alternative energy sources. Indeed, Gen4Energy estimates one of its reactors will cost $100 million to build, compared to the $4 billion to $6 billion in capital needed to build a larger conventional plant.
Once the reactor is manufactured, the SMR will be moved to site for final assembly, via truck or train, and then be installed below ground level, depending on the design. The fuel is already sealed inside the unit, so, once in place, the reactor would be able to be plugged into the local electricity network, ready to generate power. At the end of its lifetime, the entire reactor would be shipped back to the factory or a regional fuel centre and treated, removing the need to store spent fuel separately.
However, this ease of transport and accessibility does raise concerns with some groups, including Greenpeace, about security and proliferation. In response, manufacturers highlight that the reactors are easier to secure and so lessen the possibility of proliferation.
Spent fuel from SMRs is not significantly different from existing used fuel. The solutions that have been found for the existing fleet of 400 global reactors would be embraced for SMRs. The waste management, decommissioning and disposal are now considered at the outset of the design process and integrated with the build specification of the systems. For the fast neutron and high temperature SMRs, the waste management is considered at the outset as part of the overall fuel cycle system.
Gen4Energy proposes that its small reactor would actually help to prevent nuclear proliferation, as the company will handle the entire fuel cycle, including management of the irradiated fuel. Reactor cores will also be remotely monitored to prevent attempts to divert nuclear materials. Such a lease-back business model would be welcomed, for example, by developing countries wanting to acquire nuclear energy for peaceful use, but for whom it wouldn’t make economic sense to invest in the supporting fuel cycle infrastructure.
Beyond electricity
While SMRs are being considered as a ‘start-up’ option by some countries wanting to access nuclear power for the first time, other key applications exist. For example, the reactors can also generate steam for heating or to drive industrial processes in which large, traditional reactors would be too expensive and inflexible to deliver the requirements for such industrial applications.
Four such small 62 MWth units are already operating in a remote corner of the Siberian Arctic, producing steam for district heating. A similar setup could provide heat and electricity for energy-intensive operations such as oil shale recovery.
SMRs can also be coupled to desalination plants to produce fresh water for agriculture and domestic use. Fresh water supplies are reaching critical limits, with the International Atomic Energy Agency (IAEA) predicting that two-thirds of the world population will face water shortages by 2025. While more than 12,500 desalination plants exist worldwide, these energy-intensive processes are mostly driven by fossil fuels. Nuclear reactors can provide heat in a large range of temperatures, so they could easily be adapted to a desalination plant.
While SMRs offer huge potential to industry and governments worldwide to meet growing energy needs, there are design certification and licensing considerations. Their small size, transportability, siting and novel applications raise particular challenges. This is why light water-based SMRs, which are similar to the existing, already-tested traditional light water reactors in the US, are likely to be deployed first.
The future looks bright for small modular reactors. The US Electric Power Research Institute, along with the Oak Ridge National Laboratory, recently released a study that stated the US has the potential to generate 201 GWe from SMRs.
Meanwhile, the IAEA and US DoE forecast the SMR market could be worth $250 billion with up to 1,000 units built by 2050. If they are taken up on this scale then there will be a need for international cooperation and supervision to ensure the setup and disposal of the nuclear systems are carried out in a controlled and safe way.
EXAMPLES OF SMR DESIGNS
1. Light water reactors
- Babcock & Wilcox mPower reactor: 125 MWe reactor using gravity, convection and conduction to cool the reactor in an emergency
- Holtec International: 140 MWe reactor to be built underground
- NuScale Power reactor: 45 MWe that is scalable; a single facility could have up to 24 reactor modules
- Westinghouse reactor: 200 MWe, built on established concepts
2. Fast reactors
- GE Hitachi PRISM: 300 MWe reactor that can recycle spent fuel and as with some other designs would be built on seismic isolators to dampen effects of earthquakes
- General Atomics Energy Multiplier Module: 240 MWe reactor that can also convert spent fuel into electricity and industrial process heat
- Gen4Energy Hyperion Power Module: 25 MWe reactor initially designed to power processes in oil shale fields
- Toshiba 4S: 10M We reactor for use in remote locations
3. High-temperature gas-cooled reactors
- AREVA Antares: 250 MWe primarily for industrial applications
- General Atomic Gas Turbine Modular Helium Reactor: 285 MWe reactor
- Pebble Bed Modular Reactor, South Africa: 80 MWe reactor delivers super-heated steam and generates electricity
- Chinergy: 210 MWe reactor with passive safety systems
Ocean Power
The use of small modular reactors in marine propulsion looks set to rise. Lloyd’s Register recently joined forces with US-based small reactor developer, Gen4Energy (formerly Hyperion Power Generation), British vessel designer, BMT, and Greek shipping operator, Enterprises Shipping to explore marine applications for SMRs. The group is putting together a concept tanker-ship design for a 70 MWe reactor.
At the same time, Babcock International's marine division has completed a study on developing a nuclear-powered liquefied natural gas tanker, pinpointing which shipping routes and cargoes were best suited to nuclear propulsion. The study also noted that technological advances in reactor design and manufacture have made this option more attractive to shippers.
Taking the notion of ocean power a step further, Russia's Ministry for Atomic Energy, Rosatom, is building a series of floating power stations destined to power offshore oil and gas exploration as well as remote communities in the Russian Arctic. Each floating power station will be erected on a 144 m-long barge, and comprise two modified naval propulsion reactors providing 300 MWt and 70 MWe.
Refuelling will take place every three years, while every 12 years, the entire plant will be towed back to the wharf where it was constructed, for overhaul. Each reactor is destined to last 40 years, and Russian media reports claim at least seven vessels will be built by 2015.
BIOGRAPHIES
Professor Richard Clegg is the Global Nuclear Director for Lloyd’s Register. He has worked in the nuclear industry for almost 30 years, in business, academia and government. Prior to joining Lloyd’s Register, Professor Clegg was the MD of the UK National Nuclear Centre of Excellence, and before that Chief Scientist at the UK Atomic Weapons Establishment. He was the founder and Director of the Dalton Nuclear Institute at the University of Manchester, and began his career with BNFL, leaving as the Group Science Director.
Professor Mamdouh El-Shanawany is the Nuclear Technical Director of Energy at Lloyd’s Register. He was previously at the International Atomic Energy Agency where he was Head of Safety Assessment Section, the Division of Nuclear Installations Safety. Professor El-Shanawany was a member of the IAEA team that shared the 2005 Nobel Prize for Peace. Before moving to nuclear regulation he spent almost 20 years within the UK nuclear industry. He is currently a visiting professor of Nuclear Engineering at the Imperial College of Science and Technology, London University.
Keep up-to-date with Ingenia for free
SubscribeOther content from Ingenia
Quick read
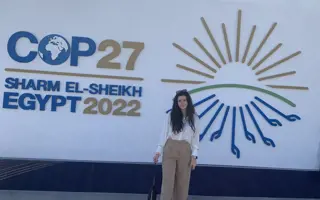
- Environment & sustainability
- Opinion
A young engineer’s perspective on the good, the bad and the ugly of COP27

- Environment & sustainability
- Issue 95
How do we pay for net zero technologies?
Quick read
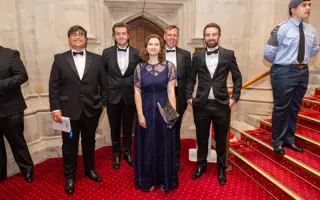
- Transport
- Mechanical
- How I got here
Electrifying trains and STEMAZING outreach
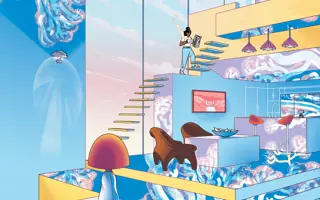
- Civil & structural
- Environment & sustainability
- Issue 95