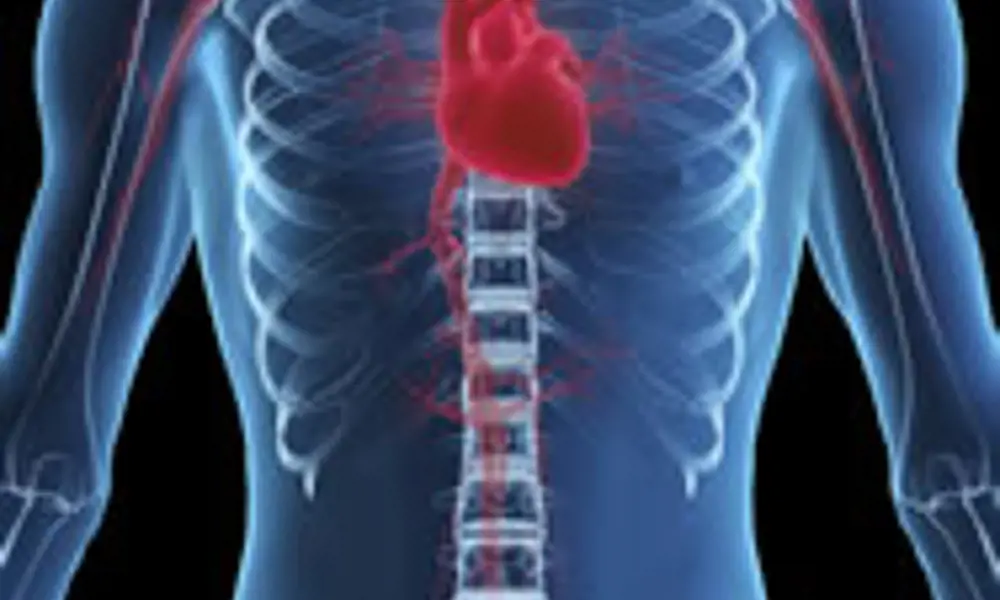
Modelling health and disease
© Mads Abildgaard
The Virtual Physiological Human (VPH) project has been created to support and progress research in biomedical modelling and simulation of the human body. This European initiative aims to improve our ability to predict, diagnose and treat disease, and have a dramatic impact on the future of healthcare. Dr Clare Sansom, a freelance science writer for Ingenia, talked to Miriam Mendes, VPH Project Coordinator, University College London and Professor Peter Coveney, Director of Centre for Computational Science at UCL.
In recent decades, more and more science and engineering projects have been ‘big science’ or ‘grand challenges’: international, interdisciplinary enterprises on a truly vast scale, costing many billions of dollars, euros, pounds or yen. It is difficult to think of a much grander challenge than building a complete, computer-based model of human physiology in health and disease, at all levels from molecules and cells to organs and systems, that is accurate enough to use for testing new pharmaceuticals. Such a project is well under way: the global Human Physiome Project (every bit as ambitious as the Human Genome Project) is tackling this engineering challenge
The European Union’s contribution to this project is an investment of approximately €200million in a “collaborative investigation of the human body as a single complex system” between 2007 and 2013 within its Framework 7 programme. Under the banner of the ‘Virtual Physiological Human’ (VPH), European-led, international research groups are building models with patient data, aimed at developing techniques for patient-specific, early diagnosis and tailored treatment. This fits in as part of the EU’s vision of enabling “personalised, predictive and preventative” healthcare by 2020.

Modelling that shows the density and organisation of collagen fibres across the wall of the heart. This information, represented by the mathematical heart model, is essential for defining the mechanical properties and electrical conductance of the model © Auckland Bioengineering Institute, University of Auckland
VPH mission
Projects within the VPH programme aim to model the human body at different scales, from the molecular (measured in nanometres) to the whole body (in metres), and from the microseconds needed for protein-protein interactions to a complete human lifespan. It does not need much imagination to see the role for engineers in the analogies of engineering processes – pushing, stretching, pumping, filtering – that abound in human physiology. Biomedical engineering is one of the key disciplines for the development of the initiative along with bioinformatics and medical informatics.
It is clearly impossible to build a model of a complete human from scratch. The VPH initiative, alongside similar projects in other parts of the world, relies on groups (always inter-disciplinary and often multinational) building models of organs and systems at different scales that can be bolted together and that may eventually – almost certainly decades hence – form a complete ‘virtual human’. Such an avatar might be used, for example, to simulate an individual patient’s condition or to develop accurate computer-based drug tests that reduce (although not completely remove) the need for experiments on animals.

The heart model is shown with a translucent outer wall and the internal (left and right ventricular) surfaces are shown in brown. The small gold coloured planes show the orientation of the myocardial sheets, on which the muscle fibre orientiation is shown as a silver line. This illustrates the changing orientation of the fibre-sheet structure of the heart © Auckland Bioengineering Institute, University of Auckland
Leading with the heart
Some organs are easier to model than others. Perhaps surprisingly, the organ that has proved most amenable to this approach is one of the most vital: the heart. Computer-based cardiac modelling has a long and distinguished history, dating from long before terms such as ‘bioinformatics’ were coined, before the UK joined the EU and only just after Watson and Crick’s DNA models set the scene for modern molecular biology.
In 1960, a young physiologist called Denis Noble, then a PhD student at University College London, showed that it was possible to use differential equations to model the movement of ions into and out of heart cells, and thus some of the minuscule electric currents that control the heartbeat. His programs, written on punched cards and run on one of the first UCL computers, gave him a first-author paper in Nature journal and arguably launched a discipline as well as a career. Noble soon moved to the University of Oxford, where he continues to build ever more sophisticated models of heart cells.
Heart-cell models today use hundreds of differential equations to model a large proportion of the myriad molecular processes that take place in these cells. There are now known to be over 50 different kinds of ion channel and pump at work in the membranes of heart cells.
Yet it was only when Noble’s group began to collaborate with a bioengineer, Peter Hunter and his colleagues at the University of Auckland, New Zealand, that the virtual heart models that are now proving useful in drug development could be conceived. Hunter’s group obtained detailed measurements of the geometry of mammalian hearts and the arrangement of their tissues, and developed a three-dimensional computer model
of heart anatomy as a “scaffold” on which Noble’s cell models could be arranged.
The model uses a relatively small number of high order finite elements to simulate the orientation of heart muscle fibres throughout the myocardium (heart muscle). Simulating the movement of electric currents through these ordered heart muscle cells produces a model of the electrical fluctuations during the normal heartbeat that is remarkably accurate. Even more remarkably, perturbing this movement – as in disease, in drug treatment or even in the normal fight-or-flight response triggered by adrenaline – causes physiologically realistic changes in the pattern of the heartbeat.
Pharma interest
This is something that the pharmaceutical industry is seriously interested in. Many drugs have failed in late-stage clinical trials, or, at an even greater cost to the companies involved in both money and prestige, have to be withdrawn from the clinic because of serious side effects in a tiny minority of patients.
Vioxx, marketed by Roche in the early 2000s as a painkiller and often prescribed for arthritis, was withdrawn from the market because of a small but significant increase in the risk of heart attack and stroke. Such problems often arise when drugs interact with one particular ion channel in heart muscle cells, causing a lengthening of part of the cardiac cycle known as “long QT syndrome”. Normally, this is harmless, picked up only in ECG traces, but in individuals who have genetic mutations that lengthen the QT interval, a further, drug-induced lengthening can trigger serious and life-threatening arrhythmias. Pharma companies now seek to identify candidate molecules that may trigger this response and remove them from their drug development pipelines at the earliest possible development stage, ideally as computer models. Noble and Hunter’s heart models are now in routine use in many companies for this purpose.
Heart models that are accurate enough to use in drug testing are impressive in themselves, but the heart functions as part of the cardiovascular system. Indeed, the body of a human (or any complex organism) is a complicated array of interacting organs and systems at different levels. Most progress has been made with heart models only because of that organ’s relative simplicity. As school-children know, the heart is a pump, and its function arises from a relatively small number of types of cells, arranged in fairly simple ways.
Most other organs, even ones that seem, superficially, to be as simple, are structurally more complex. Take the kidney: this organ can be thought of as ‘a filter’ in the same broad sense that the heart is a pump, but its basic structural element, the nephron, is made up of a more complex structure and more types of cells than the muscles of the heart wall.
The human kidney is made up of approximately one million nephrons that operate as a coordinated group with a variety of control systems associated with each nephron and within groups of nephrons. This complexity is a real engineering challenge and requires comprehensive knowledge of the structure and function of the nephrons and their constituent cell types and also of the control systems that enable this highly parallel filter to match its activities to the requirements of the body.
This section of the VPH effort is being addressed internationally by development of integrated cellular, nephron and whole kidney models in Melbourne, by scaling up the parallel computation of individual nephron function using supercomputer programming and architecture to simulate whole kidney function. The modelers utilise algorithms that grow the vasculature and nephrons according to the structure of the mammalian kidney.
Our knowledge of the kidney physiome lags a long way behind that of the cardiac physiome for two reasons: kidney research is less well funded than heart research, but, just as crucially, it is harder to model the kidney.

Simulating the entire kidney on a BlueGene/P project. In the image created by the project, the blue structures are veins and the red, arteries. The outer layer is the cortex, the next layer (pale pink) is the outer medulla, the next (central yellow area) is the inner medulla and the ureter (also yellow) can be seen at the lower left © Andrew Bonollo
Intricate jigsaw
Noble and Hunter have both been prominent movers in setting up the multidisciplinary, international collaborations such as the VPH that it is hoped will, decades hence, join the dots on modelling the whole of human physiology. As Secretary-General and, later, as President of the International Union of Physiological Sciences, Noble took the lead in launching the Physiome Project, which is a key international partner of, and complements, the VPH programme. The University of Auckland, where Hunter is based, was the only non-European founding member of the VPH Network of Excellence, one of two mechanisms through which the European Commission funds physiome-related research.
This Network of Excellence is coordinated from the Department of Chemistry in University College London, Noble’s alma mater, and is led by Peter Coveney, who stresses the powerful, integrated computational resource that the project requires: “The multi-scale modelling approaches being pursued by the VPH community implicitly require access to a vast range of resources of different scales, from the humble desktop workstation to the mightiest supercomputers. UCL has worked hard to ensure that VPH scientists have access to these resources on demand, without worrying them about the details and policies that can constitute a barrier to researchers working across disparate infrastructures.”
Researchers at any academic or not-for-profit institution that is involved in human physiome-related science or engineering may apply for general membership of this Network of Excellence. This entitles them to apply for small pump-priming grants and attend conferences, networking events and training courses. However, the lion’s share of the EU’s VPH funding is directed into large collaborative VPH Initiative projects chosen through a competitive grant application process. And it is very competitive: the last round of VPH large-project funding in 2010 attracted over 100 applications of which only 12 were successful.
These large research projects cover a variety of organs and systems in health and disease. Not surprisingly, five of the first 15 to be funded deal with aspects of the cardiovascular system. Much of the research is deliberately clinically led, with clinicians joining scientists and engineers in many of the consortia. Four projects are concerned with cancer, developing models that will aid its early detection, diagnosis and treatment. Another uses modelling to develop methods for the early diagnosis of Alzheimer’s disease. Many of the models are developed using data from individual patients and aim to stratify disease.
Fresh modelling initiatives
Engineering is important at some level in almost all of these projects. For example, in atherosclerosis, fatty deposits build up on the walls of arteries. It is not serious in itself until those deposits are thick enough to block blood vessels. Any medical intervention involving the cardiovascular system, even the simple surgical procedure of inserting a stent, will change the dynamics of blood flow, potentially changing the distribution of atherosclerotic plaques and the likelihood of severe complications. The ARTreat project led by a Greek software company, SWORD Technologies SA (see ARTreat project), aims to develop patient-specific computer-based models of arterial anatomy, blood flow and blood cell dynamics in order to predict the effect of a particular intervention on a patient’s atherosclerosis and aid clinical decision-making.
Fractured bones arising from osteoporosis, a degenerative condition in which bone density is lost, now cost European countries more than €30 billion a year. The VPHOP (Osteoporotic Virtual Physiological Human) project, coordinated from Istituto Ortopedico Rizzoli in Italy (see VPHOP), aims to develop models of osteoporotic patients that can be used to predict the risk of fracture. These are multi-scale models that take into account factors ranging from the average physical load on the whole body to the composition and function of the bone matrix, which is defined at molecular level.
Just as the human body is made up from an enormous range of components, so the Virtual Physiological Human – if it is to stand any chance of mirroring the ‘real thing’ – must be built up from an enormous number of component models at different scales. Even modelling a single organ, such as the heart, will involve a large number of groups with different approaches. Eventually all these will have to be combined. Such integration can fail for the simplest of reasons, for example, different groups can use different names for the same component. One of the most important tasks of the VPH network is the unglamorous one of building a set of common, publicly available tools, and working with people who develop standards and nomenclatures that ensure that, as far as possible, all models are compatible with each other.
If the dream of the virtual human comes to pass, when our children and grandchildren visit their doctors later this century, they may encounter avatars of themselves, tailored to mimic their exact physiological condition, and on which doctors can test preventative and curative treatments in advance. And if the virtual human does come to the doctor’s surgery, it will owe much to the insights of the young Denis Noble and his colleagues, working at the dawn of the computer age. It may, however, owe more to those who work behind the scenes now to construct the standards and software that will ensure that the disparate parts of this ambitious project will, eventually, work together.
For more information about the VPH project visit: http://toolkit.vph-noe.eu/
Keep up-to-date with Ingenia for free
SubscribeOther content from Ingenia
Quick read
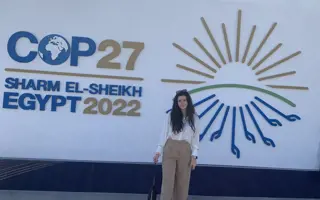
- Environment & sustainability
- Opinion
A young engineer’s perspective on the good, the bad and the ugly of COP27

- Environment & sustainability
- Issue 95
How do we pay for net zero technologies?
Quick read
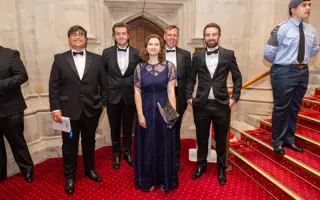
- Transport
- Mechanical
- How I got here
Electrifying trains and STEMAZING outreach
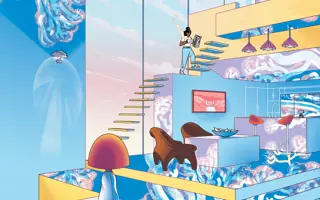
- Civil & structural
- Environment & sustainability
- Issue 95