Issues
Issue 49
December 2011
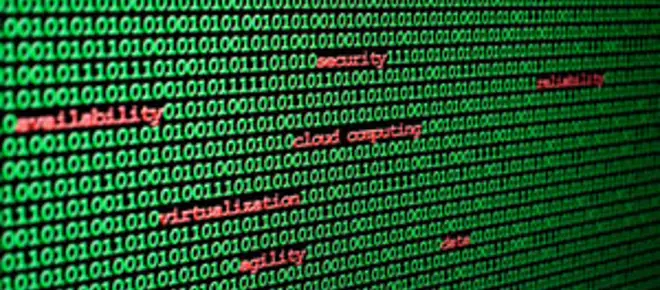
- Issue 49
Cloud computing
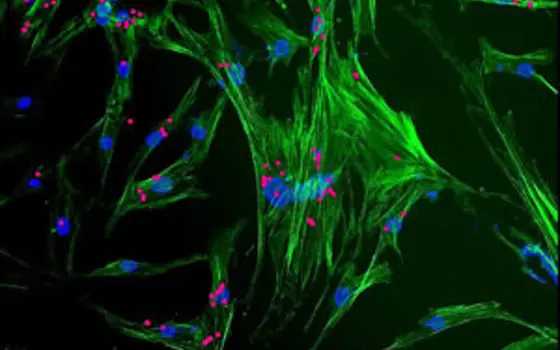
- Issue 49
Engineering cells to grow tissue
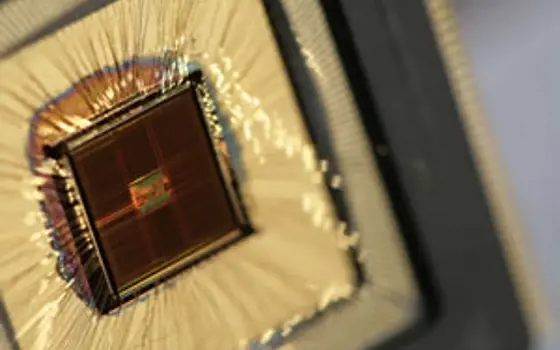
- Issue 49
Future for mobile handsets
- Issue 49
HOW DOES THAT WORK? - 3D Vision
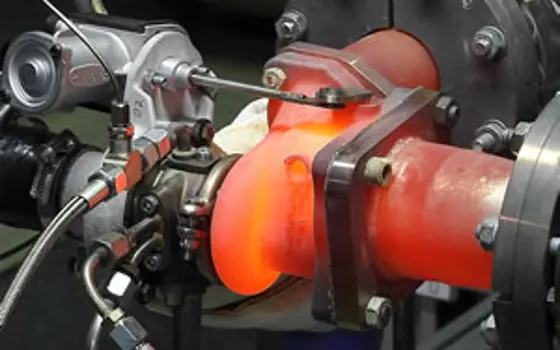
- Issue 49
How low can we go?
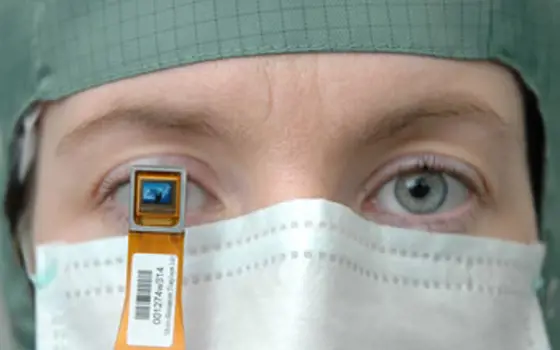
- Issue 49
INNOVATION WATCH - Indoor positioning system
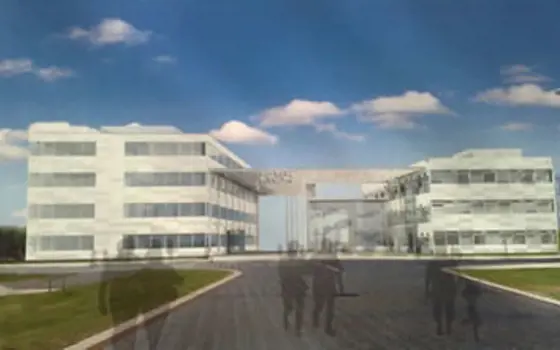
- Issue 49
Jeni Mundy FREng - Mobile systems specialist
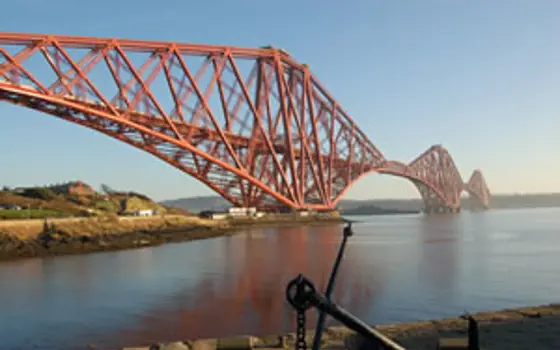
- Issue 49