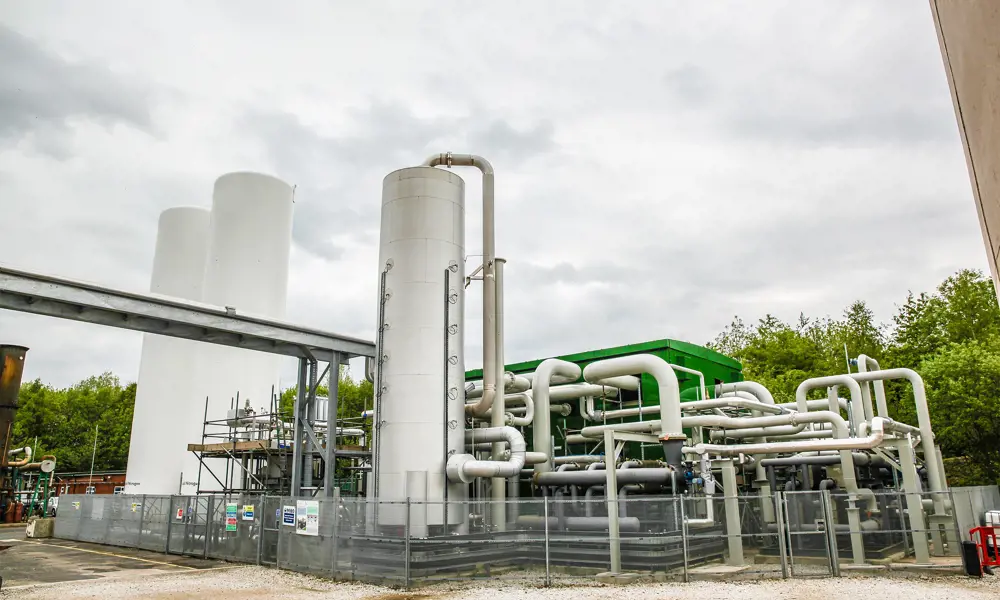
A new contender for energy storage
In years to come, 2018 may be seen as the year renewable energy came of age. At the beginning of the year UK government data showed that, for the first time, the 29% of electricity produced by wind and solar was more than the total produced by nuclear (21%). The increase means that low carbon energy sources now account for over half of all electricity generated.
However, a long-running challenge has been how to store energy at times when there could be shortfalls caused by the wind not blowing or the sun not shining. UK energy storage enterprise, Highview Power, is going some way to solve this problem. In 2018, it opened the world’s first grid-scale cryogenic (liquid air) energy storage plant in Bury. The plant has a capacity of 5 megawatts and can store 15 megawatt hours (MWh) of electricity, which is enough to power about 5,000 homes for around three hours.
Storage contenders
The main energy storage providers are pumped hydroelectric – 95% of global capacity – and lithium-ion batteries, which have been the fastest growing and account for most of the rest of the world’s energy storage. However, although pumped hydro has a long lifecycle of between 40 and 50 years and very large storage capacity, its main drawback is geographical, requiring the correct topography and two large reservoirs, the building of which can impact heavily on the environment.
In 2017, the potential of lithium-ion batteries captured the headlines when Elon Musk made a $50 million bet on Twitter that Tesla could build a 120 MWh battery storage in South Australia in under 100 days – if not, then the company would charge nothing for its construction. The state had been crippled by power shortages and took him up on his wager. Tesla delivered in 60 days and the result is the largest battery storage facility in the world, which supports and stabilises existing electricity supplies in the state.
However, although lithium-ion batteries seem to be the main contender for solving the renewable energy storage conundrum, they do have drawbacks. Lithium is relatively rare and its supply is controlled, in effect, by a handful of mining companies. Cobalt, which is needed for the cathode, is both toxic and rarer still. Although battery prices have dropped recently and they are a quick-responder when needed, batteries have relatively short lifecycles (between five and ten years), they degrade and they have a realistic limit of about four hours for harnessing large amounts of power.
Highview Power’s cryogenic storage solution ticks many boxes including sustainability, cost, effectiveness and the fact that it has a small footprint and can be sited anywhere
Highview Power’s cryogenic storage solution ticks many boxes including sustainability, cost, effectiveness and the fact that it has a small footprint and can be sited anywhere. The only box it had not marked until now was demonstration at large scale. Now, with plans to begin building two large cryogenic energy storage plants in 2019, that is set to be ticked too.
Clean cold beginnings
Highview Power has created a whole new industry – clean cold. Co-founder Peter Dearman had an idea to build a reciprocating engine that was effectively driven by liquid nitrogen and could be used to power transport. In 2005, Dearman and power researcher Toby Peters founded Highview and tested the concept at the University of Leeds. In 2011, the engine part of the business subsequently branched off to form the Dearman Engine Company and has since found applications with transport refrigeration units and other cool-power projects.
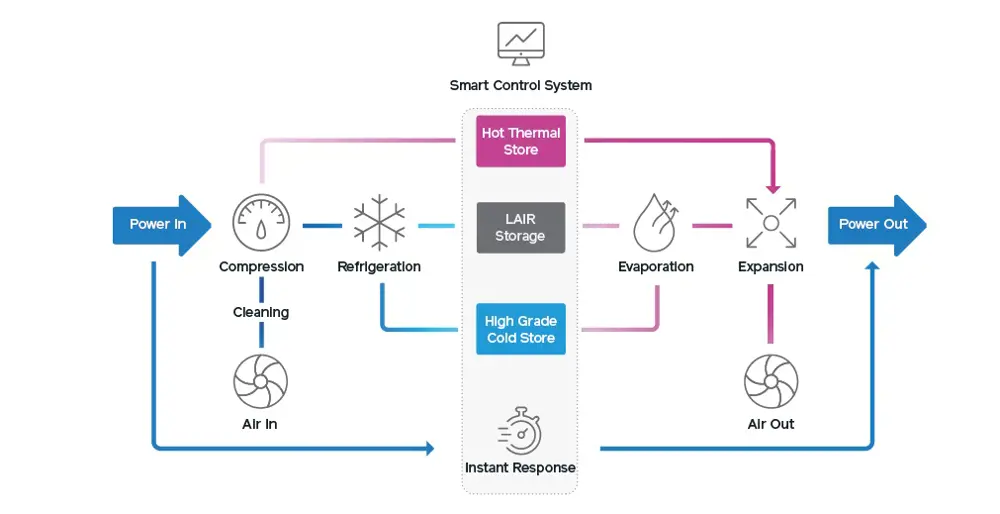
A process diagram showing the three stages of the producing, storing and recovering liquid air for generating power © Highview Power
In 2006, Gareth Brett, an electrical engineer with a background in utilities and banking, joined Highview and fundraised for a pilot plant. In 2009 he became CEO. By 2011, the world’s first liquid air pilot installation was installed in Slough and connected to the grid with the help of a £1.1 million grant from the then Department for Energy and Climate Change. The plant had a 350-kilowatt charge with two and a half megawatt hours of storage. Now the technical fine-tuning of the cryogenic storage project could be done, ensuring that an actual plant would perform in the same way as the process model of the system.
The company partnered with Professor Yulong Ding, who in 2014 was appointed as the Highview Power/Royal Academy of Engineering Research Chair in energy storage at the University of Birmingham to further develop the cryogenic energy storage technology, alongside other thermally based concepts. He had worked with Highview since 2005 and had seen the potential for energy storage at very large scale. From 2006 to 2007, Professor Ding worked with Highview to invent and file patents for cryogenic energy storage and generation. Professor Ding’s work helped develop the world’s first research centre for cryogenic energy storage at the University of Birmingham.
Utilising the claude cycle
🗘 The process that Highview is using to store power
Invented in 1902, the Claude Cycle has enabled production of industrial quantities of liquid nitrogen, oxygen and argon. It developed and improved the Hampson-Linde cycle, patented seven years before, which had introduced regenerative cooling and enabled the low temperatures required to liquefy gases. The main drawback with the Linde method had been the loss of temperature at the end of the expansion process. Georges Claude developed a cycle that used a turbo-expander to expand gas to lower temperatures for more efficient cooling. A turbo-expander operates in the same way as a turbine, expanding high-pressure gas so that it becomes lower in pressure and temperature and power can be recovered. A turbo-expander is used when its primary purpose is to cool gas, while a turbine is used when the purpose is to recover power.
Highview Power’s system has adapted both the Claude and Linde cycles. Its charging process involves the compressing of air to ~ 12 bar (a unit of pressure), before it is cleaned and compressed further to about ~ 60 bar. The air is then fed into the top of the heat exchanger in the system’s ‘cold box’, where it is cooled to around -100°C. At this point, some of the air is then bled off and expanded through a turbo-expander to cool it down further.
The remaining air that was not bled off continues through the heat exchanger. When it gets to about -180°C, it is expanded through a Joules-Thompson (JT) valve. It is at saturation point after it has finished expanding and a large portion of it condenses into its liquid phase. The remainder is a very cold gas.
The liquid and the gas enter a phase separator, which reduces the pressure, along with the air from the turbo-expander. Here, the liquid drops out and is sent to tanks, while the gas returns through the heat exchanger to the top of the system, cooling the incoming air along the way. Once it emerges from the heat exchanger, a compressor recycles it and returns it to ~ 60 bar before it rejoins the input air and forms a semi-closed loop.
Some of Highview’s patents cover the capturing of high-grade cold and recycling it into the liquefier, an important step that doubles the efficiency of the liquefier and makes it economically viable.
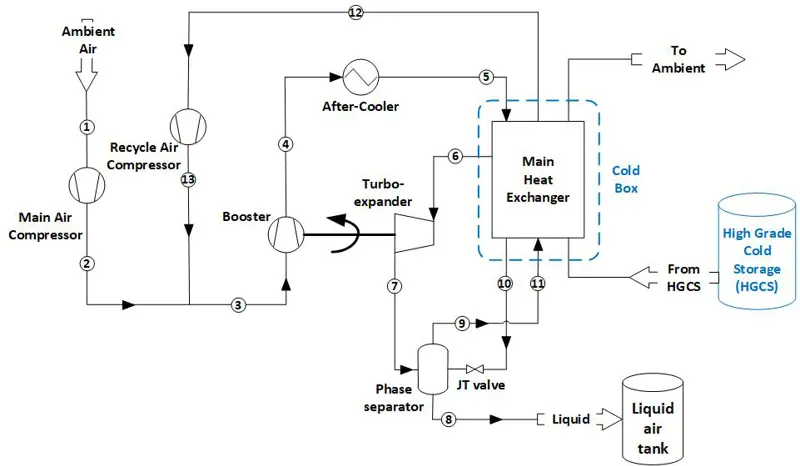
Diagram showing how Highview’s pilot plant uses the Claude Cycle. In addition, the system uses recycled cold (shown in blue) input from its cold store. (The main air compressor, the circulating air compressor and booster, the compressor for compressing the main stream air into the cold box (heat-exchanger)) © Professor Yulong Ding
How it works
The cryogenic energy storage system consists of three main components: a charging device that uses off-peak or excess electricity to power an industrial liquefier to produce liquid air; an energy store where the liquid air is held in an insulated tank at low pressure, and a power-recovery unit where re-gasified liquid air is used to drive a turbine and generate electricity.
Ambient air is taken from the surrounding environment then using electricity it is cleaned, dried and refrigerated through a series of compression and expansion stages until the air liquefies. This process is based on a modified version of the Claude Cycle and can convert 700 litres of ambient air into one litre of liquid air [see Utilising the Claude Cycle].
The liquefied air – air turns to liquid when refrigerated to a sufficiently low temperature
(~ -196°C at atmospheric pressure) – is kept in insulated tanks (acting as giant thermoses) at low pressure, which function as energy stores. When power is required, liquid air is withdrawn from the tanks, pumped to high pressure, reheated and then expanded. The resulting high-pressure gas is then used to drive expansion turbine generators to generate electricity. No fuel is burnt in the process, which results in the exhaust producing clean dry air.
Operating efficiency is enhanced by adding two stores. The first is a thermal store that gathers the compression heat generated during air liquefaction and is used for increasing air temperature prior to expansion in the turbine. The second is a proprietary cold store that captures waste cold from the regasification of liquid air while power is recovered and later delivers the cold into the liquefier to reduce power consumption – these measures close the thermal loop for cryogenic energy storage [see Cold storage technology and materials].
The separation of the power, storage and recovery components provides flexibility in the system’s design. Because power and energy are independent of each other, requirements can be tailored so that clients can decide how much of a tank they want to fill, how much energy to store and then how quickly it needs to be emptied. This modularisation of the process enables different configurations and solutions to suit each customer.
Cold storage technology and materials
🧊 Ensuring strength, energy density and high charge and discharge speeds
High-grade cold storage plays a key role in reducing the power consumption of the air liquefaction process and hence improving the round-trip efficiency of cryogenic energy storage. The first consideration to make when compiling the liquid air system’s components was the selection of appropriate cold storage technology. Packed-bed (a hollow tube, pipe or other vessel that is filled with a packing material) was chosen because it serves as both a cold storage device and a heat-exchanger. This selection was based on the technology’s maturity, cost, performance and scalability.
The other major selection considerations were to do with the cold storage materials. The density and specific heat capacity of cold storage materials need to be as high as possible to increase the energy density of the store and reduce the physical size – and therefore cost – of the containment structure.
The material’s thermal conductivity should be sufficiently high to ensure that the charge and discharge speeds can be achieved quickly. It should also be able to minimise the temperature gradient inside the individual particles to ensure efficient heat transfer.
The material’s mechanical properties needed to be strong enough to withstand thermal stresses due to the system’s repeated thermal cycling. It was also required to cope with the hydrostatic stress due to packing. After much testing, gravel was chosen as the cold storage material. The gravels picked had a thermal conductivity of ~ 1.8 W/m.K, specific heat capacity of 0.71 kJ/kg.K and a density of 2600 kg/m3. The added advantages of the gravel were its low cost and the fact that it could cope with both ambient and cryogenic temperatures.
Demonstration plant
High-grade cold storage plays a key role in reducing the power consumption of the air liquefaction process and hence improving the round-trip efficiency of cryogenic energy storage. The first consideration to make when compiling the liquid air system’s components was the selection of appropriate cold storage technology. Packed-bed (a hollow tube, pipe or other vessel that is filled with a packing material) was chosen because it serves as both a cold storage device and a heat-exchanger. This selection was based on the technology’s maturity, cost, performance and scalability.
The other major selection considerations were to do with the cold storage materials. The density and specific heat capacity of cold storage materials need to be as high as possible to increase the energy density of the store and reduce the physical size – and therefore cost – of the containment structure.
The material’s thermal conductivity should be sufficiently high to ensure that the charge and discharge speeds can be achieved quickly. It should also be able to minimise the temperature gradient inside the individual particles to ensure efficient heat transfer.
The material’s mechanical properties needed to be strong enough to withstand thermal stresses due to the system’s repeated thermal cycling. It was also required to cope with the hydrostatic stress due to packing. After much testing, gravel was chosen as the cold storage material. The gravels picked had a thermal conductivity of ~ 1.8 W/m.K, specific heat capacity of 0.71 kJ/kg.K and a density of 2600 kg/m3. The added advantages of the gravel were its low cost and the fact that it could cope with both ambient and cryogenic temperatures.
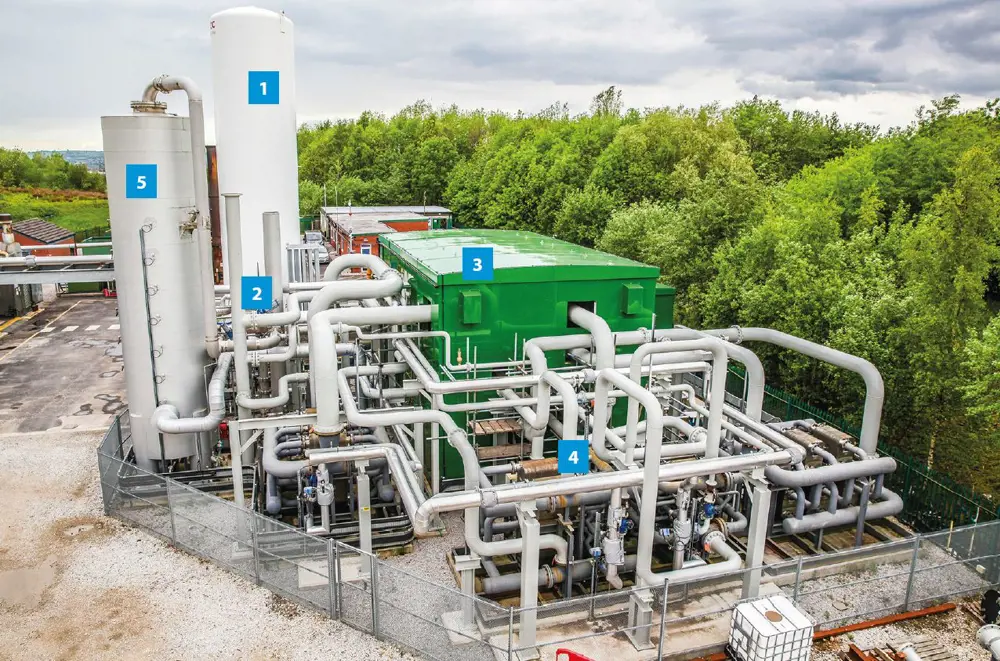
1. Liquid air storage 2. Cryo pumps 3. Power turbine and generator (5 MW ) 4. Heat exchanger containers 5. High grade cold stores © Highview Power
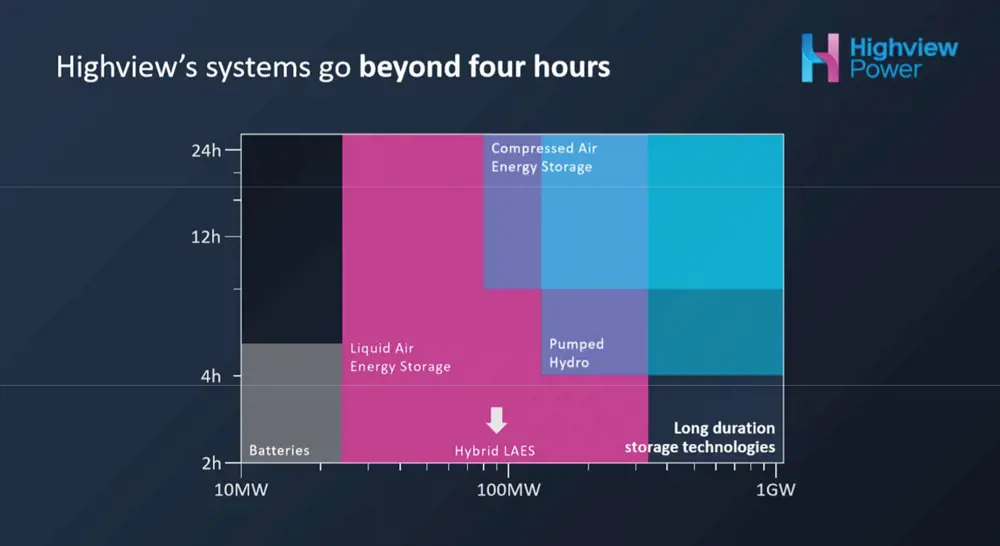
A diagram showing the energy storage options available in terms of time and capacity © Highview Power
The energy storage equipment that the company incorporates is already globally used for the bulk storage of liquid nitrogen, oxygen and LNG – such as heat exchangers, packed-bed storage units and cryogenic insulation. This way it can confidently state the lifespan and efficacy of each plant’s hardware – Highview will guarantee a 30-year lifetime for its systems. The end-of-life recycling characteristics of the system are also favourable as most of the tanks and equipment are made of stainless steel with some copper in the generators; apart from lubricating oil, Highview states that the process uses nothing toxic.
The last piece of the jigsaw to be fitted before the first big sale was to improve the response times when supplying energy to the grid. For this purpose, supercapacitors and flywheels have been added to the Pilsworth plant and response times are now measured in seconds.
Highview Power sees cryogenic energy storage as being most useful in supplying the electricity grid via reserve, regulation and balancing. The idea of reserve is to keep spare capacity in the system and keep a cache of stored energy. The company can scale-up and incorporate three or four extra tanks of stored energy for this purpose. Effectively, balancing is about moving energy from one time slot to another. Regulation entails keeping the frequency constant so that the plant is matching in real time, second by second, supply with demand – stabilising frequency.
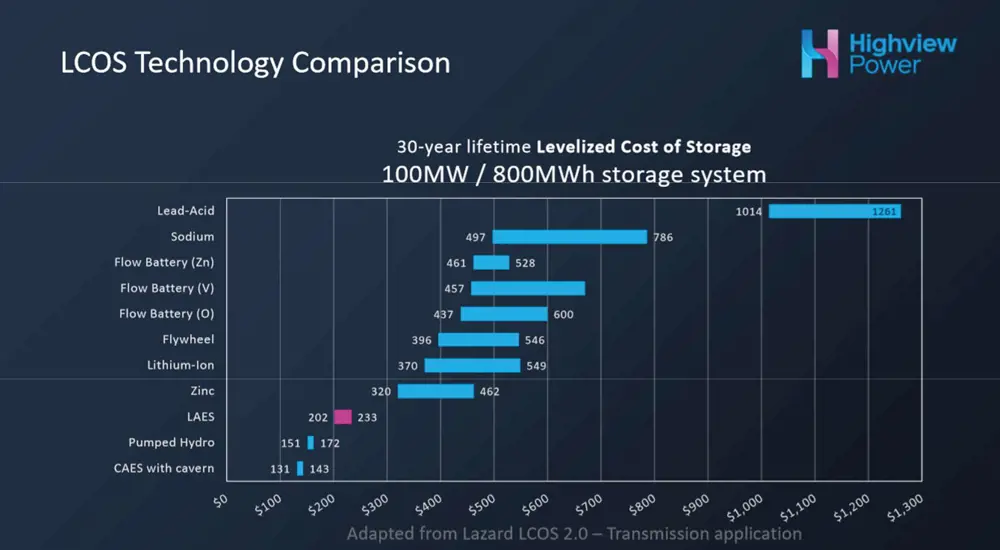
This chart shows the cost of cryogenic energy storage (LAES) provided by Highview Power’s system over 30 years compared to other forms of energy storage © Highview Power
Why now?
In September 2018, Dr Javier Cavada was appointed as CEO and he sees the future storage capacity of liquid air increasing from five hours to perhaps 20 hours and then a couple of days or even weeks. Liquid air can be kept for months until eventually the air in it boils off and then must be topped up with liquid air; usually, the system cycles daily and never reaches this point. He also feels that the 50 megawatt size will, thanks to the modularity of the system, increase to 100 megawatt. In five years’ time, he thinks that there will be around 20 Highview cryogenic energy storage plants around the world supplying and storing 10 gigawatt-hours of energy.
Long-duration storage has always been critical to enable the wider deployment of renewable energy and overcome the intermittent nature of solar and wind energy. It seems that cryogenic energy storage can provide that and will soon be smoothing out the peaks and troughs of renewable demand in the UK and wherever it is needed.
***
This article has been adapted from "A new contender for energy storage", which originally appeared in the print edition of Ingenia 78 (March 2019).
Contributors
Dominic Joyeux
Author
Dr Javier Cavada is president and CEO of Mitubishi Power and a Board Director of Galp. At the time of publishing, he was CEO and President of Highview Power. He joined the company in 2018 after 17 years in leadership positions at Wärtsilä Corporation, including executive roles in China, Italy, the Netherlands, Spain, and Finland. He completed a PhD in industrial Engineering at the University of Cantabria.
Gareth Brett is the technical consultant at Highview Power. At the time of publising, he was Chief Technology Officer at Highview Power. He joined the company in 2006 and helped lead it in taking cryogenic energy storage from concept to market-ready technology. From 2009, he served as CEO before being appointed Vice Chairman and Chief Technology Officer in 2018.
Professor Yulong Ding is the Director of Kelvin Thermotech and a Professor of Chemical Engineering at the University of Birmingham. At the time of publishing, he was the Director of the Birmingham Centre for Energy Storage. In 2014, he was appointed as the Highview Power/Royal Academy of Engineering Research Chair in energy storage at the University of Birmingham. He also holds the founding Chamberlain Chair of Chemical Engineering at the university.
Keep up-to-date with Ingenia for free
SubscribeRelated content
Energy
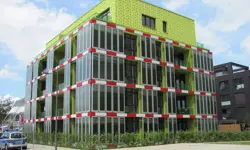
Algae-powered architecture
An apartment block in Hamburg in Germany has been built that uses microalgae placed within its façade to generate heat and biomass. Jan Wurm, an associate director at Arup, was one of the chief designers of the energy system. He talked about the concept, execution and results from the world’s first photobioreactor.
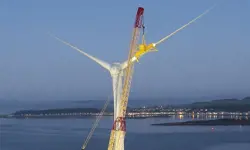
Digital hydraulics for wind energy and beyond
Research that has helped change the technology for harnessing wind energy has many other applications. The digital hydraulics system devised by Artemis Intelligent Power has received many accolades, the latest being the winner of the 2015 MacRobert Award.
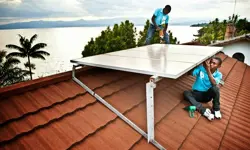
New energy pioneers
London-based BBOXX supplies solar-powered battery boxes to customers in developing countries. Their remote monitoring and battery management system was one of the winners of the 2015 Bloomberg New Energy Finance Award.
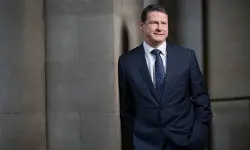
Energy with connections
When Steve Holliday FREng moved from the oil industry into energy distribution, the sector was seen as staid. In reality, during his years at National Grid, the sector became increasingly important as the need to tackle climate change led to a transformation in the UK’s energy mix.
Other content from Ingenia
Quick read
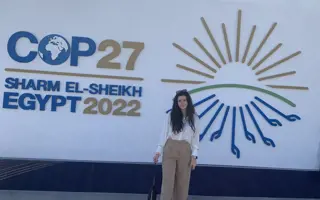
- Environment & sustainability
- Opinion
A young engineer’s perspective on the good, the bad and the ugly of COP27

- Environment & sustainability
- Issue 95
How do we pay for net zero technologies?
Quick read
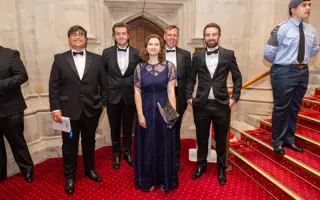
- Transport
- Mechanical
- How I got here
Electrifying trains and STEMAZING outreach
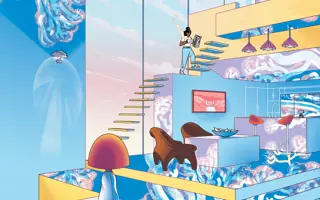
- Civil & structural
- Environment & sustainability
- Issue 95