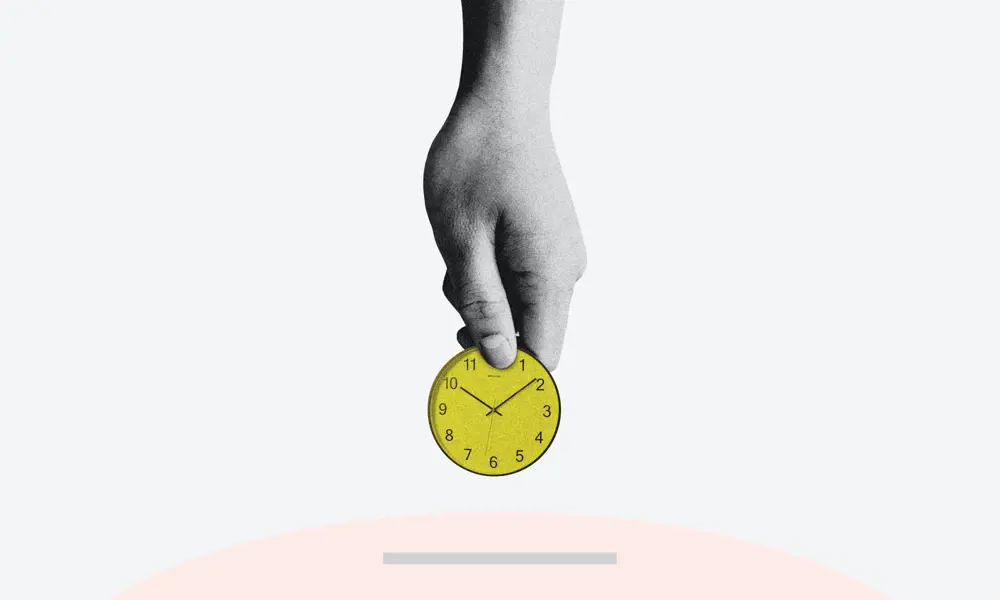
Why microseconds matter
You wake up in the morning as your alarm on your smartphone goes off, synchronised to your wake-up time by a network of satellites (‘Supercharging GPS precision’, Ingenia 90) overhead. The very same technology tracks your location on your morning run. You boil the kettle to make a cup of tea with electricity from the grid – which is itself synchronised by satellite-based timing. On the platform at the train station, you see on the overhead signs that the train is delayed by one minute, thanks to coordination of its GPS location with your local station.
We unknowingly reap the benefits of technologies that can precisely detect time and location numerous times a day, every day. Even just on our smartphones, we have a direct line to this invisible yet integral utility that underpins much of the infrastructure and systems that keep the world turning. It has been estimated that satellite-based positioning, navigation and timing services – the global navigation satellite systems (GNSS), such as GPS – directly support over £250 billion (13.4%) of the UK economy. As a result, a breach or failure in GNSS can cause all manner of problems with services we rely on, not to mention potentially costing the UK economy over £1 billion a day.
We have come to rely so deeply on these technologies that the resilience in the provision of timing is now a challenge.
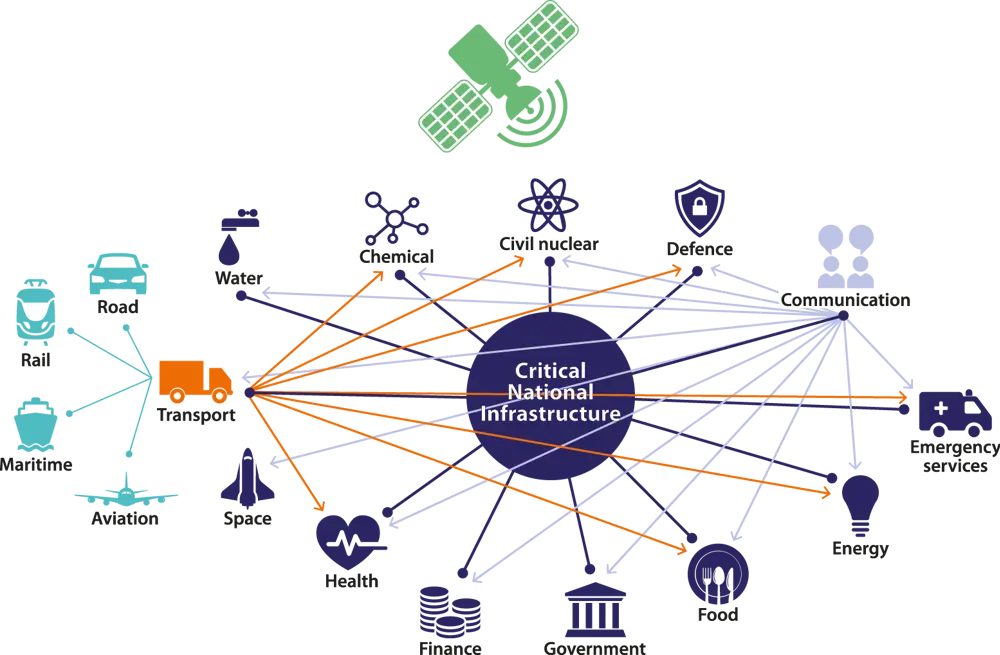
Critical national infrastructure describes the resources, systems, processes, and facilities that keep society and the economy functioning, from generating electricity and transmitting it around the country, to producing and transporting food in agricultural supply chains. The communications networks and transport systems that underpin so many other sectors are heavily reliant on GNSS (adapted from a diagram provided by the National Physical Laboratory)
Measuring time with atoms
A few thousand years ago time was simply measured by light and dark and the movement of astronomical bodies across the sky – all you needed to know was when to get up to labour in the fields and when to stop and go to bed.
Before clocks as we know them came along, humans devised ingenious methods to measure the passing of time, from falling sand in an hourglass, the slow burning of incense, or the flow of water between vessels. Water clocks became increasingly sophisticated in 11th-century China and the medieval Islamic world, with geared mechanical systems displaying different mannequins on the hour.
Whether for religious prayer or widening webs of commerce, as societies became more interlinked and synchronised across geographies, timekeeping became increasingly sophisticated. Between the 15th and 20th centuries, the world saw the development of striking clocks in bell towers, spring-based watches, much more accurate pendulum clocks, and electric and quartz oscillator clocks. In tandem came new learnings in science and technology: from measuring gravity and modelling the shape of the Earth, to locating ships at sea.
Two timing innovations, in particular, are cornerstones of the world today.
One is the quartz oscillator, invented at Bell Laboratories in 1927. Based on the electronic oscillations of a quartz crystal, it is used in the majority of the world’s clocks, watches, computers, and devices such as kitchen timers. While more accurate than its predecessors, the frequency of oscillation depends on the temperature and dimensions of the crystal, so no two quartz clocks are the same. For most purposes, calibrating against another time reference – solar time, or the Earth’s rotation period – was sufficient.
However, because of the Earth’s fluctuating spin, solar time and the ‘true’ universal time gradually deviate from one another (which also causes strange side-effects, such as the leap second – see box-out). For scientists chasing this ‘true’ measurement of time, an alternative benchmark was needed.
A breach or failure in GNSS can cause all manner of problems with services we rely on, not to mention potentially costing the UK economy over £1 billion a day.
In 1955, physicists at the UK’s National Physical Laboratory (NPL) built an atomic clock based on counting energetic transitions in caesium atoms (‘Compact atomic clocks’, Ingenia 57). It quickly became apparent that atomic time was a much more stable and consistent benchmark than the Earth’s rotation, which led to a new definition of the second in SI (Systeme Internationale) units in 1967. Today, the successors of these clocks sit in national laboratories the world over, from Teddington in the UK to Washington in the US, and are used to calculate the Coordinated Universal Time (UTC) standard time scale.
NPL has managed the UK’s time scale for many decades at its site in Teddington. The time scale is based on a suite of hydrogen masers and caesium beam clocks, steered by caesium fountains that provide the realisation of the SI second.
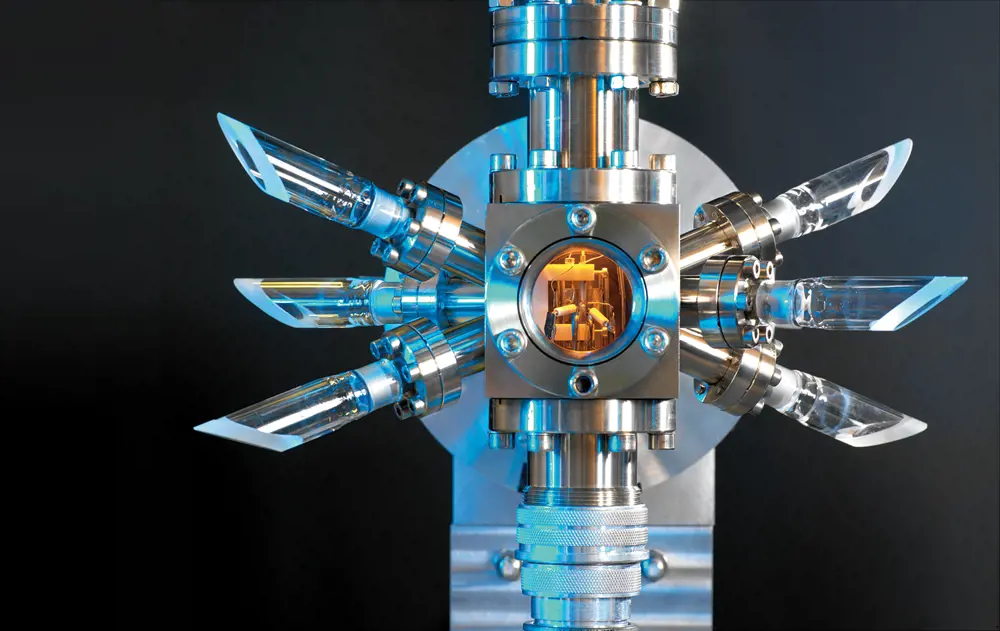
The next generation of atomic clocks at the National Physical Laboratory, using laser‑cooled trapped ions or atoms, should achieve accuracies about 100 times better – equivalent to gaining or losing no more than one second in the age of the universe © National Physical Laboratory
The leap second
And how it brought down Reddit and LinkedIn
In 1972, physicists introduced the leap second as a way to ‘correct’ UTC to solar time: meaning that every few years, one extra second has been added to the end of a day. Since then, 27 leap seconds have been applied to UTC. However, recently, technology companies such as Meta and Google have been arguing that the leap second should be phased out with an alternative, because it can cause computer programs to crash and, historically, outages on some of the world’s most popular websites, including LinkedIn and Reddit. Moreover, in the last few years, the Earth has seen more than 30 of the shortest solar days since high-precision measurement of time began, with about a millisecond and a half shaved off each of them. If solar time continues to run faster than universal time for long enough, a negative leap second will be required. With as-yet unknown effects, it could cause even more chaos in the tech world than its positive counterpart.
What is universal time?
Atomic clock data from about 75 timing laboratories around the world, such as NPL, is used to define UTC. All clock data is submitted to the Bureau of International Weights and Measures (BIPM) in France. Every month, UTC is computed as an average of all the data received and published in a report called Circular T.
The upshot is that most national time scales operate a few nanoseconds faster or slower than UTC. This drift can occur because of electronic or gravitational effects, for example, and can vary over the course of a year. Time scale laboratories can correct this drift, or ‘offset’, to UTC, in a process called ‘steering’. This is usually done by shifting the frequency of the output – rather than the clock itself – with a device called a frequency offset generator. (Alternatively, the lab can accept the offset and inform their user community accordingly, who can then account for it within their own systems.)
One of the most important ways UTC is used is for GNSS – see ‘Supercharging GPS precision’ in Ingenia 90 for more. While atomic clocks are found in orbit onboard GNSS satellites, the timing signals that they use are steered by clock facilities on the ground. The latter tend to be steered themselves to time scales managed at national facilities so that they remain close enough to UTC. For example, the GPS ground segment clocks at Shriever Air Force Base are steered to the UTC time signal from the US Naval Observatory in Washington DC. The result is that the time provided by GPS is within 40 nanoseconds of UTC.
Timing in GNSS satellites
How it works
Position and time are obtained by a GNSS receiver using time-of-arrival measurements of timing signals from a minimum of four satellites. The fourth satellite is needed to determine the time offset between the receiver’s internal clock and GNSS system time to measure 3D position coordinates and time. This enables the receiver to correct its internal clock, now steered to the signals from space, and effectively offer atomic clock timing capability. The use cases resulting from this invisible utility touch all areas of our daily life, from our morning alarm clock to our train journey home.
Keeping the radio on and electricity flowing
The importance of precise and accurate timing manifests in surprising ways. For example, the slightest timing anomaly can disrupt entire TV and radio networks. In January 2016, GPS receivers skipped by just 13 microseconds and caused disturbances across UK digital radio services for several hours. The same event also caused police and fire radio equipment in parts of North America to stop working.
From live sport to primetime TV shows, broadcasting big events also depends on large audio and video data files synchronising between the filming venue and the processing studio to provide a harmonised audio-visual experience for viewers. Because large chunks of data are transferred separately, and recombined after transfer, the traceability of the time source is vital. Accurate time enables data integrity, analytics, governance, timestamping, and latency monitoring, for a seamless viewing experience.
Timing is also essential for the energy grids supplying homes and buildings with electricity. To balance electrical loads and avoid outages, grid operators must increase or decrease the electricity supply produced by generators. To do this, they need to know the currents at different locations, with very accurate timestamps. Phasor measurement units (PMUs) can be used to do this: they take real-time measurements of alternating current (AC) waveforms on the energy grid. They sample the AC waveform (at the microsecond level of accuracy) across sections of the grid and typically receive their time signals from GNSS systems. Many PMUs also have atomic clocks as a backup in case of GNSS outages.
Synchronisation and PMUs are starting to be used more widely as we get our energy from a wider variety of sources. Whether the source is a conventional power station, a wind farm, or consumers selling electricity back to the grid (for example if they have their own solar panels), a more intelligent grid will be key to a reliable supply. With the growing complexities of our energy supply and demand, PMUs could in future be used to help automate load balancing. However, this means the energy system could become vulnerable to GNSS outages unless alternative timing approaches are used.
Trading at (almost) light speed
Accurate and traceable time also keeps the finance sector ticking along. Stocks and futures exchanges can take place in less than the time it takes to click a mouse – milliseconds or even nanoseconds for some high-frequency trades. High-frequency traders are in a ‘race to zero’: making transactions faster using every possible means. Every millisecond and microsecond is vital. For example, to communicate between different financial centres, microwave links, where data travels through the air, are preferable to optical fibres. In optical fibres, the information is coded in a light beam before travelling along a glass fibre, where it is slowed to two-thirds of the speed of light in a vacuum. (One emerging approach to better this involves hollow-core optical fibres, in which light has been shown to travel at 99.7% of its speed in a vacuum – see ‘Hollowing out a future in fibre optics’, Ingenia 79.)
At these speeds, it is vital that data on these transactions is correctly timestamped and synchronised to a universal reference time. Knowing precisely when every trade takes place is essential to properly regulate trading. For example, in a matter of minutes in 2010, Wall Street’s Dow Jones index temporarily lost almost 9% of its value. Stock values dropped by over $850 billion, although luckily, mostly recovered by the end of the day. Although there are different theories to explain this so-called ‘Flash Crash’, the lack of synchronised timestamping for transactions at the time meant that proper analysis is impossible, even now.
Accurate and traceable time also keeps the finance sector ticking along. Stocks and futures exchanges can take place in less than the time it takes to click a mouse – milliseconds or even nanoseconds for some high-frequency trades.
Market regulators have now wised up to this, with a 2018 European regulation, known as MiFiDII, setting much tighter requirements for timestamping trades. For human trading (such as over the phone or online), it states that accuracy must be within one second. For automated trades performed by algorithms, it states accuracy must be within one millisecond of UTC for electronic trading, and just 100 microseconds for high-frequency trading. Trading organisations face fines of up to five million euros, or 10% of global turnover, if they don’t meet these standards. (Outside of Europe, the international finance regulator, IOSCO, has also put forward a recommendation for global markets to implement UTC-traceable timestamps for trading.)
To adhere to MiFiDII, GNSS is used for timestamping by many financial organisations. However, it can be spoofed or jammed (see box-out). It can also be vulnerable to poor signal or errors, such as that might result from a solar storm. One solution for this available to UK traders is NPLTime®. Accurate to a microsecond, its time signal – directly traceable to UTC – is delivered via a fibre-optic cable.
Fortifying our time services
As we have seen, much of our critical infrastructure relies on precise timing – and in particular, many of these pillars of society are dependent on GNSS. Interferences or outages could bring down these very pillars, with potentially life-threatening effects. This might mean preventing the emergency services from locating emergencies or sending a plane off its scheduled route and creating the potential for a collision. Clearly, there is a need for alternative technologies that can reduce our dependence on these weak signals from space. Furthermore, since the time scale delivered by NPL operates from a single site, it can be thought of as a single point of failure – if it breaks down, there is no backup.
The UK is making steps towards a more resilient national time scale by establishing the National Timing Centre (NTC) programme. During the five-year programme, a new geographically distributed national time scale will be developed by NPL and a UK-wide team of researchers, situated on several secure sites connected to one another. Rather than choosing only one method for transferring time and frequency signals between sites, resiliency will be achieved through diversity. What this means is using several different methods of transfer, such as fibre, satellite communications and even GNSS, each with very different failure modes. Known as the Resilient Enhanced Time Scale Infrastructure (RETSI), it will be a world first, and form the core of a systems-of-systems approach to national timing resiliency.
Spoofing and jamming
Disruptive interference of GNSS signals can occur unintentionally or maliciously, with several instances of hostile state actors demonstrating this capability over the past few years. Potential interferences include jamming or denial of service, rebroadcasting a GNSS signal intentionally or accidentally, or spoofing GNSS signals to create a controllable misreporting of position or time. Jamming devices, for example, are easily available online, and can be used by car thieves to secretly prevent a driver from locking their door. However, many systems that rely on signals from GNSS have procedures in place to deal with any GNSS-based system faults, and some infrastructures use backup timing devices such as atomic clocks that offer business continuity for a duration before drifting out of specification.
The NTC programme, rolling out systems over the next two years, aims to ensure split-second timing is available wherever it is needed, whether on our devices or in the energy, broadcast or finance industry. More resilient timing systems that are independent of GNSS will for one thing, enable faster, more secure internet. But even more importantly, they will help society weather solar storms and resist jamming, from the emergency services to the London Stock Exchange – making the potential havoc that we might see today a thing of the past.
***
This article has been adapted from "Why microseconds matter", which originally appeared in the print edition of Ingenia 92 (September 2022).
Contributors
Dr Leon Lobo is Head of the National Timing Centre (NTC) programme at NPL, focusing on developing and delivering a national timing strategy.
Keep up-to-date with Ingenia for free
SubscribeRelated content
Aerospace
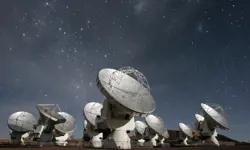
ALMA – the high altitude observatory
The Atacama Large Millimetre/submillimetre Array (ALMA) is the largest and most expensive ground-based telescope built, revolutionising our understanding of stars and planetary systems. Building it in the Atacama Desert in Chile required the ingenuity of hundreds of engineers.
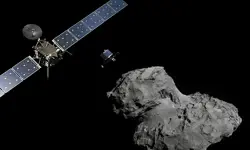
Communicating with outer space
The Royal Academy of Engineering awarded a team at BAE Systems the Major Project Award in June 2016 for their development of a powerful satellite modem system, pivotal in enabling the precise control of the pioneering Rosetta spacecraft and the first-ever soft landing of a spacecraft on a comet.
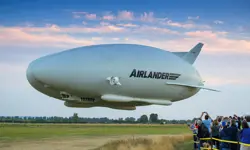
An aircraft like no other
The Airlander made headlines when it embarked on its first test flight in August 2016 as the world’s largest aircraft. Chris Daniels at Hybrid Air Vehicles Limited, and David Burns, Airlander’s Chief Test Pilot, talk about the engineering that helped it reach this stage and plans for the craft’s future.
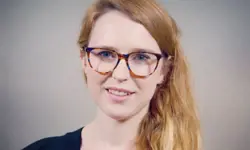
Q&A: Lucy Harden
Lucy Harden is a mechanical engineer on BAE Systems’ Digital Light Engine Head-Up Display development programme. She devises innovative solutions for pilots to display essential flight information that sits directly in their line of sight and is overlaid onto the real world.
Other content from Ingenia
Quick read
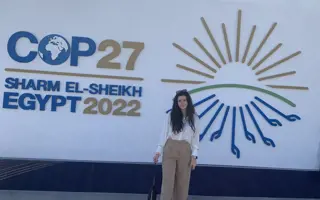
- Environment & sustainability
- Opinion
A young engineer’s perspective on the good, the bad and the ugly of COP27

- Environment & sustainability
- Issue 95
How do we pay for net zero technologies?
Quick read
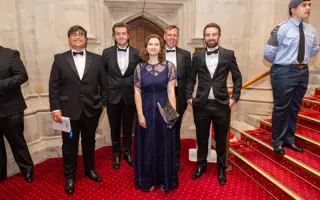
- Transport
- Mechanical
- How I got here
Electrifying trains and STEMAZING outreach
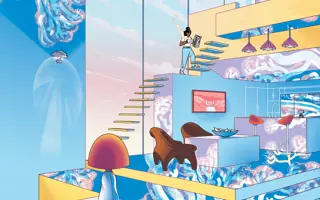
- Civil & structural
- Environment & sustainability
- Issue 95